- Academic Editor
This is an open access article under the CC BY 4.0 license.
Background: Continuous glucose monitoring (CGM) has the potential to be a valuable tool for measuring glucose concentrations in preterm neonates, but its actual effect on infants is still unclear. Therefore, we conducted a meta-analysis to evaluate the clinical effect of CGM on blood glucose levels in preterm infants requiring intensive care. Methods: We searched PubMed, Embase, CINAHL, Web of Science, Cochrane Library, and Cochrane Database of Systematic Reviews for randomized controlled trials (RCTs) comparing CGM with other interventions, and identified five studies that met our eligibility criteria. The quality of the included studies was assessed using Cochrane’s Collaboration tool. Results: Our meta-analysis demonstrated that CGM, when combined with a protocol for adjusting glucose infusion, was associated with a decrease in the average duration of hypoglycemia, a greater percentage of time spent in the euglycemic range, and reduced time spent in mild and severe hypoglycemia compared with other interventions and controls. Conclusions: Our findings suggest that CGM, with a protocol for adjusting glucose infusion, increases the time spent in the euglycemic range, and reduces the duration of hypoglycemia in preterm infants during the first week of life.
Premature neonates are predisposed to glucose control challenges, particularly within the initial weeks of life. They manifest a proclivity for hypoglycemic episodes, alongside the emergence of hyperglycemia attributed to insulin resistance [1, 2]. In newborns, poor glucose management is linked to poor neurodevelopmental outcomes, chronic brain damage, retinopathy, sepsis, intraventricular hemorrhage, and mortality [3, 4, 5, 6].
As with hyperglycemia, hypoglycemia, and glycemic instability, premature babies had higher risk of death and morbidity in a period after birth [7, 8, 9]. Hyperglycemia can induce osmotic diuresis and metabolic acidosis, and it has been associated with an increased susceptibility to intraventricular hemorrhage, patent ductus arteriosus, retinopathy of prematurity, necrotizing enterocolitis, and white matter loss [10, 11, 12]. Attempts to limit hyperglycemia hazards can raise the risk of hypoglycemia, which has been linked to poor developmental outcomes [13, 14].
However, achieving normoglycemia in premature infants proves challenging due to the diverse interplay of insulin resistance, insulin insufficiency, and inadequate energy reserves on neonates [13]. In addition, investigations utilizing masked subcutaneous continuous glucose monitoring (CGM) have revealed protracted periods of both hyperglycemia and hypoglycemia that were clinically silent but were linked to poor developmental outcomes in children [15].
Therefore, enhancing early glucose management might be a significant modifiable risk factor for preterm baby outcomes. Real-time CGM, which measures CGM and detects early patterns in glucose concentrations, has been effectively employed in adult and pediatric critical care settings to support clinical choices. The aim of this paper is to review the actual clinical benefits on continuous, real-time, glucose monitoring in preterm infants, and the lack of up-to-date evidence.
The study included randomized controlled trials (RCTs) and survey studies comparing glycemic variability, blood glucose levels and data of related blood glucose. Participants who received CGM and combined with a protocol for adjusting glucose infusion were classified as intervention group. Also, participants who received other intervention were classified as control group.
The electric signal is transmitted via radio frequency to an external monitor,
which aggregates the signal at five-minute intervals and converts it into glucose
levels, generating a dataset of 288 interstitial glucose concentration points per
day. The guidelines outlined advised implementing insulin or additional dextrose
support. In scenarios where capillary blood glucose levels ranged between 50 and
60 mg/dL, the intravenous glucose supply was augmented by 1 g/kg/day, and the
subsequent glucose level was assessed after a 2-hour interval. Instances of
hypoglycemia (
The primary outcome measure was the time spent in euglycemic range, presented as percentage (the time spent in euglycemic range/total time).
The second outcomes were incidence of hypoglycemia and average duration of the hypoglycemia, incidences of hypoglycemia are presented by times per day, and average duration of the hypoglycemia are presented by minute.
The databases MEDLINE/PubMed, Cochrane Library/Embase, CINAHL, Scopus, Web of Science, and ProQuest were searched for relevant studies published from their inception dates up until 30 October 2021, using various key terms, such as CGM, neonatology, critical care, and hypoglycemia. Additional papers were found by manually checking reference lists from included trials, (systematic) reviews, meta-analyses, and health technology evaluation reports. Only items that were published in English were considered.
Two authors (WL & YH) independently read the titles or abstracts of every record obtained to find research that needed to be evaluated further. All possibly relevant articles were read in their entirety. In situations where only abstracts were accessible, we attempted to locate the trials’ final publication. Studies that did not result in a final publication of the findings were evaluated independently. We sought to optimize the quantity of information by evaluating all accessible data at the same time in situations where multiple publications and reports accompanied primary research.
The inclusion criteria were: (1) RCTs or survey studies; (2) preterm infants
with gestational age at birth
The exclusion criteria were that studies were eliminated if accuracy estimates could not be extracted either directly from the paper or from information given by the authors.
The study selection was carried out separately by two authors (WL and YH). Any disagreements were settled via consensus. Fig. 1 shows the modified PRISMA flow chart for research selection [16]. The study was registered in the International Prospective Register of Systematic Reviews (PROSPERO; ID: CRD42021286541).
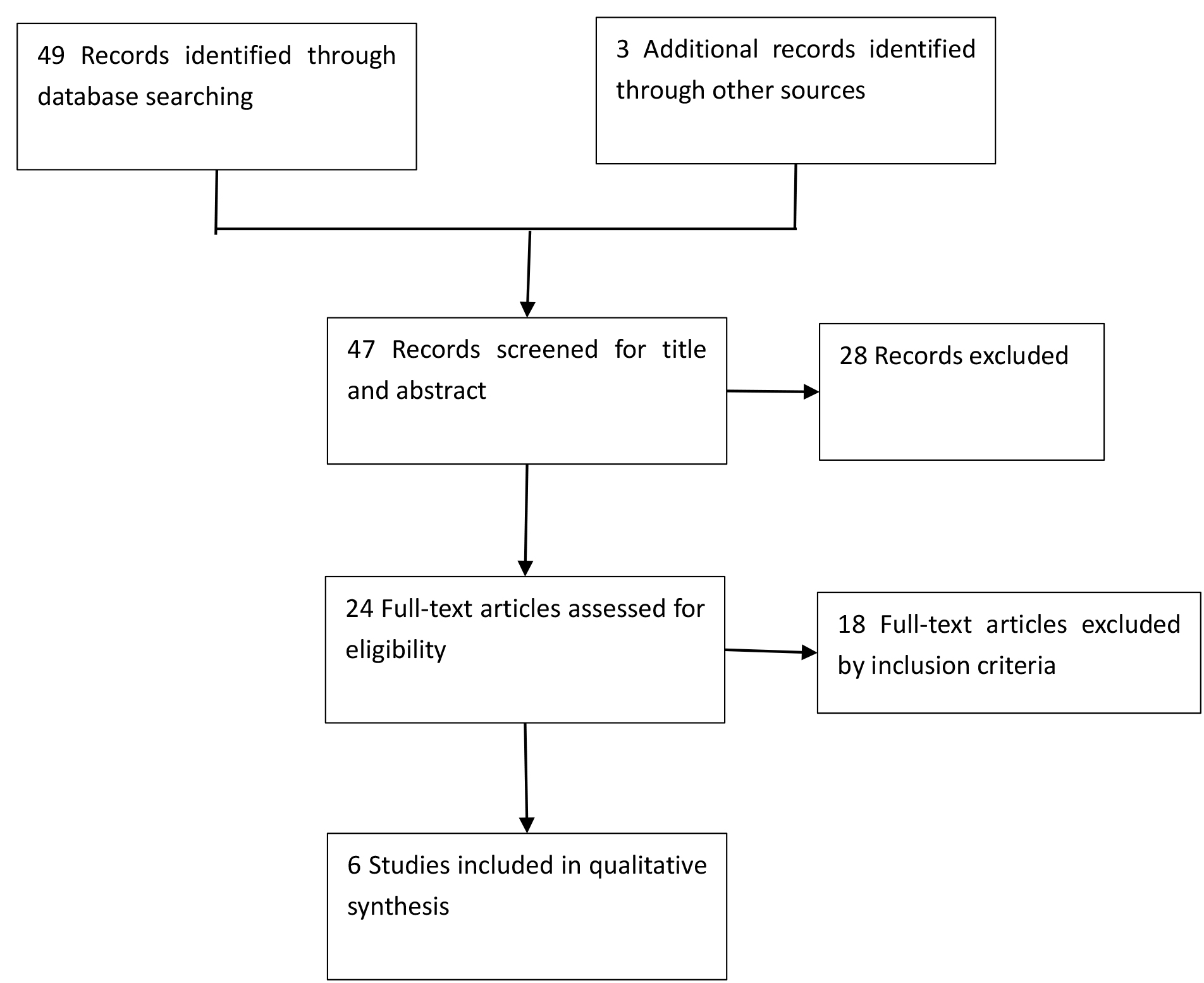
Literature search and study selection process.
Three authors (WL, YH, and YC) independently extracted the relevant population and intervention characteristics from studies that met the inclusion criteria using standard data extraction templates. The original authors of the paper were contacted for any missing essential information. The extracted data are shown in Table 1 (Ref. [17, 18, 19, 20, 21]).
Study | Sample size | Gestational week (week) | Birthweight (g) | Maternal diabetes | Age of study participants | Threshold for hypoglycemia | Threshold for hyperglycemia | Other intervention |
Beardsall, 2021 [17] | IG: 70 | IG: 910 (160) | √ (n = 13) | standard care | ||||
CG: 85 | CG: 880 (180) | |||||||
Thomson, 2019 [18] | IG: 10 | 26.14 (1.9) | IG: 901 (144) | - | standard care | |||
CG: 10 | CG: 823 (282) | |||||||
Beardsall, 2020 [19] | IG: 10 | 27.0 (2.4) | IG: 962 (142) | - | a paper algorithm | |||
CG: 10 | CG: 823 (282) | |||||||
Galderisi, 2017 [20] | IG: 25 | IG: 30 (29–31) | IG: 1170 (1100–1595) | √ (n = 5) | without CGM | |||
CG: 25 | CG: 30 (28–31) | CG: 1300 (1100–1760) | ||||||
Uettwiller, 2015 [21] | IG: 22 | IG: 29.6 (24.1–34.7) | IG: 1014 (579–1485) | √ (n = 2) | - | intermittent capillary glucose testing | ||
CG: 21 | CG: 30.1 (24.4–37) | CG: 1000 (620–1485) |
IG, intervention group; CG, control group; CGM, continuous glucose monitoring.
Two authors (WL and YH) independently assessed the risk of bias for each study by the Quality Assessment of Diagnostic Accuracy Studies-2 tool. For each outcome, the Grading of Recommendations Assessment, Development, and Evaluation process was used to determine the overall level of certainty of the evidence.
We used random-effects model to estimate effect size between intervention group participants and control group participants. Significant was defined as a p-value of less than 0.05. We used SPSS 22.0 (SPSS, Chicago, IL, USA) to execute statistical analysis.
We imputed standard deviation (SD) from other studies if the SD is missing [22]. A meta-analysis was carried out in order to compute a weighted average of the overall mean differences from the various studies included in the model. To compare the different in outcomes of interest in response to CGM and other intervention, a forest plot was used. We used Revman 5.3 (The Nordic Cochrane Centre, Copenhagen, Denmark) for building meta-analysis and forest plots.
The literature searches run in October 2021 identified 49 references. Two authors (WL and YH) assessed 24 full-text articles for eligibility. 18 of these studies were excluded because they did not meet the eligibility criteria.
Five RCTs recruiting 293 preterm infants met the inclusion criteria [17, 18, 19, 20, 21]. We have listed the details of these trials in the characteristics of included studies section (Table 1). Three studies compared the use of CGM to standard care to measure glucose blood levels [17, 18, 21]. In one study, the use of CGM associated with prespecified interventions to correct hypoglycemia or hyperglycemia, was compared to CGM without such prespecified interventions [19]. Also, one study used intermittent modalities to measure glucose blood levels [20], and one study used intermittent capillary glucose testing to complete measure [21].
Beardsall et al., 2021 [17] included 155 preterm infants who within 24 h
of birth, had a birthweight of 1200 g or less, and had a gestational age of up to
33 weeks plus 6 days. Compared with infants in the standard care group, CGM group
had more proportion of time in euglycemic range than that of the standard care
group. There were no serious adverse events related to the use of the device or
episodes of infection. The real-time CGM system utilized in the study comprised
an Enlite
Thomson et al., 2019 [18] included 20 preterm infants within 48 hours from birth. In the study, real-time CGM combined with a paper guideline to target glucose control was compared with standard neonatal care (masked CGM). Percent time in target range was greater with CGM than point-of-care (POC) blood glucose. The use of CGM was perceived as sufficient utility in preterm infants. Real-time CGM was performed using the Paradigm® Veo.
Beardsall et al., 2020 [19] included 20 preterm infants with a birth
weight
Galderisi et al., 2017 [20] included 50 preterm infants with
Uettwiller et al., 2015 [21] included 43 preterm infants with
The collective study quality is visually represented in Fig. 2 (Ref. [17, 18, 19, 20, 21]). Notably, the general quality of the studies was found to be somewhat constrained. The encompassed investigations demonstrated an absence of high risk of bias across the assessed domains of the Cochrane “Risk of Bias” tool, except notably for blinding of participants and personnel. A majority of the studies exhibited a low susceptibility to selection bias and reporting bias.
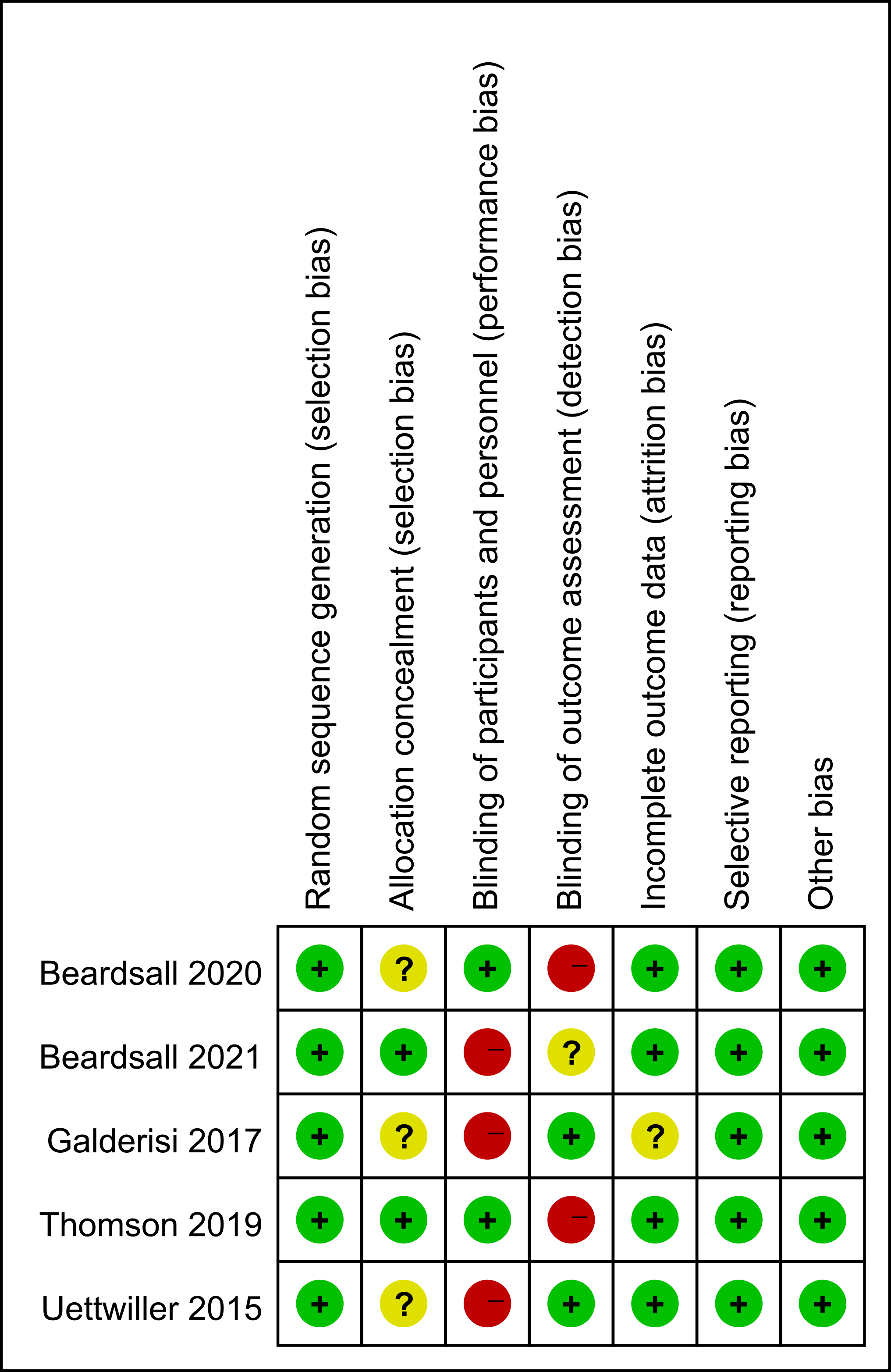
Risk of bias summary.
Five studies [17, 18, 19, 20, 21] reported this outcome. Based on subgroup analysis of
percentage of time spent, whether the mother had diabetes or not, the preterm
infants who received CGM had more proportion of time in euglycemic range than
that of other intervention in the first week of life. This included diabetic
mothers (percentage mean of time spent: 24.2%, 95% confidence interval (95%
CI) 15.7 to 32.7, n = 269, p
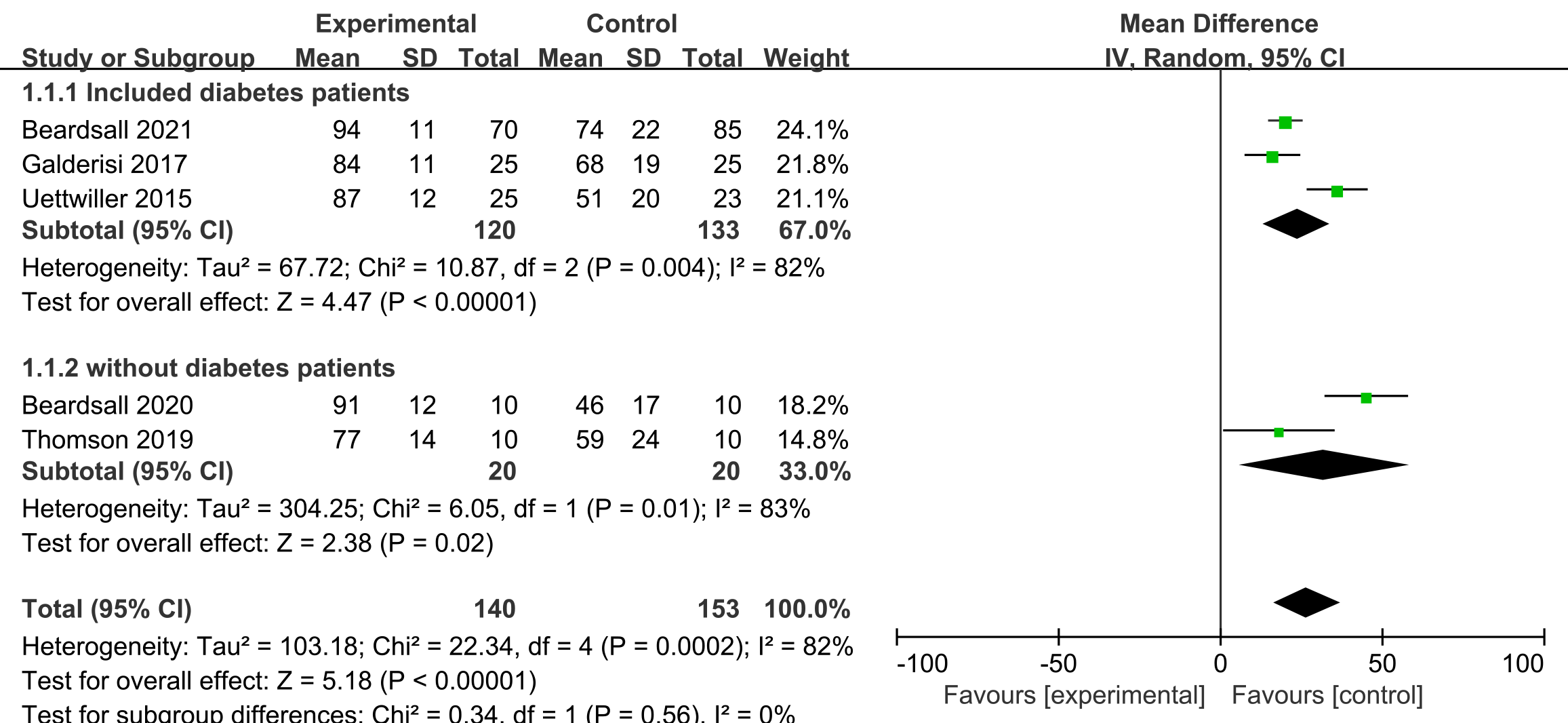
Forest plots of proportion of time in euglycemic range of CGM and other intervention in preterm infants. SD, standard deviation; 95% CI, 95% confidence interval; CGM, continuous glucose monitoring.
Two studies [17, 19] reported on incidence of hypoglycemia, and no difference
was observed in its incidence, when comparing CGM and other interventions
(

Forest plots of incidence of hypoglycemia in preterm infants (times per day).
Four studies assessed the different effect between CGM and other intervention on
average duration of the hypoglycemia [17, 18, 19, 20]. Compared with other interventions,
the participants with CGM had shorter duration of hypoglycemia during the study
period, and the duration of the hypoglycemia between two interventions was
significantly different (mean duration 90.7 min, 95% CI –204.2 to –122.8, n =
245, p

Forest plots of average duration of the hypoglycemia in preterm infants (min).
From the rigorous evaluation of this systematic review and meta-analysis, it becomes evident that CGM exhibits a substantial efficacy in maintaining euglycemic range in preterm infants, thus contributing effectively to a reduction in the duration of hypoglycemia. However, our research found no discernible impact of CGM on the incidence of hypoglycemia. These findings convey the safety and necessity of CGM implementation for patients at high risk of hypoglycemia.
Our data, in concurrence with the emerging body of literature, reinforces the potential of CGM in mitigating the duration of hypoglycemia. The overall quality of the evaluated material was moderate, with minor uncertainties regarding application. Our meta-analysis revealed that CGM can proficiently stabilize the blood sugar levels in premature infants, thus increasing the proportion of time in the euglycemic range when the preterm infants were hypoglycemia. However, the applicability of CGM in providing similar glycemic control in newborns with various conditions remains unknown. Furthermore, the uncertainty surrounding the risk of hypoglycemia can be attributed to the utilization of various CGM devices. Real-time CGM offers insights into dynamic blood glucose fluctuations, whereas retrospective CGM holds the potential for more accurate blood glucose monitoring. In the various RCTs incorporated into our meta-analysis, CGM demonstrated superior glycemic control in preterm infants compared to intermittent capillary blood glucose testing and standard care, yet the proportion of time spent in the euglycemic range varied significantly among preterm infants with different conditions. Our results also indicate a reduced duration of severe hypoglycemia with CGM usage. The efficacy of CGM in controlling various levels of hyperglycemia and hypoglycemia warrants further investigation. Two studies [17, 20] reported similar results, benefits, and potential risks of CGM usage in preterm newborns. The efficacy of CGM in controlling is currently under review in a Cochrane systematic review, albeit without considering diagnostic glycemic control effects [23].
Significant therapeutic effect is warranted on maintaining a normal blood sugar range in premature infants when the patient is in hypoglycemia. Anthropometric improvements affirm the original motivation for the development of diagnosis and treatment strategies for premature infants. Furthermore, we delineate the demographics of patients who may benefit from glycemic control. Given the significant data supporting improved hypoglycemia treatment outcomes, particularly for premature infants with parents who have diabetes or related genetic diseases, CGM appears to be a promising optimization strategy.
CGM holds substantial potential as a diagnostic instrument within neonatology; however, its routine adoption is impeded by notable constraints. Technical intricacies linked to sensor placement and calibration, along with the distinctive physiological attributes of neonatal glucose metabolism, present hurdles in achieving precise and consistent monitoring. The absence of standardized reference values for neonatal glucose levels adds complexity to the interpretation and establishment of intervention thresholds. Moreover, ethical considerations and patient safety mandates the necessity of comprehensive attention prior to the integration of CGM into routine neonatal care. The imperative of surmounting these challenges via heightened research and developmental efforts cannot be understated, as it holds the key to refining the applicability of CGM within neonatology and enhancing patient outcomes.
A recent systematic review [24] examined the accuracy of CGM in preterm infants. Their results indirectly suggested that CGM could stabilize blood glucose through more accurate glucose monitoring. However, their study did not explore the actual impact on patients, thus serving as a single piece of evidence supporting the efficacy of CGM in stabilizing blood glucose levels in preterm infants.
A paramount strength of our study resides in the meticulous application of Cochrane methodologies, which facilitated an exhaustive and systematic exploration of the literature landscape. By adopting these methodologies, we have effectively mitigated the potential for reviewer errors and biases. Additionally, our approach encompassed the utilization of a tailored threshold to discern and eliminate disparate thresholds that could have contributed to the observed heterogeneity.
Certain limitations must be acknowledged when interpreting the conclusions of this systematic study. The lack of a representative research population, a reference standard, and critical information about the CGM device itself, cautions against overinterpretation of these results. Given that CGM is a still-evolving technology, significant variations exist among studies in terms of CGM brands, calibration processes, and data analysis platforms.
In future research, we should further explore the sensitivity effect of CGM on different degrees of premature birth.
The outcomes derived from this systematic review and meta-analysis serve as a foundational basis for substantiating the efficacy of CGM in expediting the resolution of hypoglycemic episodes among premature infants. Despite certain limitations and variations in study methodology, the results suggest that CGM could be a promising tool for effective glycemic control, particularly in high-risk populations. However, further research is warranted to assess the effectiveness of CGM across diverse conditions and levels of hyperglycemia and hypoglycemia. The implications of these findings hold potential for optimizing clinical strategies and improving outcomes for premature infants, especially those with a family history of diabetes or related genetic diseases.
All data generated or analyzed during this study are included in this published article.
The authors confirm contribution to the paper as follows: study conception and design: WL, YH; data collection: WL, YH, YC; analysis and interpretation of results: WL, YH; draft manuscript preparation: WL, YH, YC. All authors reviewed the results and approved the final version of the manuscript.
Ethical approval was obtained from the ethical committees of the First Affiliated Hospital of Jinan University, Guangdong, People’s Republic of China (KY-2023-175). Informed consent from all subjects was obtained at the Department of Prenatal Diagnosis of the First Affiliated Hospital of Jinan University. Patient records/information were anonymized and deidentified before analysis. All methods in this study were performed in accordance with the Declaration of Helsinki and relevant regulations.
The authors thank staffs of the First Affiliated Hospital of Jinan University for device application support.
This research received no external funding.
The authors declare no conflict of interest.
Publisher’s Note: IMR Press stays neutral with regard to jurisdictional claims in published maps and institutional affiliations.