- Academic Editors
Background: Environmental endocrine disruptor-diethylhexyl phthalate (DEHP) or its active metabolites-mono(2-ethylhexyl) phthalate (MEHP) has the greatest endocrine disrupting potency. The present systematic review and meta-analysis was to investigate the effects of DEHP/MEHP exposure on the folliculogenesis and ovarian steroidogenesis in female rodents. Methods: A search was conducted using EMBASE, PubMed, Web of Science, and Cochrance Library databases. The meta-analyses were performed using mean difference (MD) and random-effects model. Risk of bias and subgroup analyses were assessed using Revman 5.4.1 and R 4.1.2. Registration number: PROSPERO CRD42021292264. Results: A total of 15 studies were included in this systematic review. We found that the exposure of DEHP/MEHP significantly increased the ovary weight (p = 0.003), decreased the serum progesterone levels (p = 0.0008) and delayed the vaginal opening (p = 0.01). Conclusions: The DEHP/MEHP exposure has adverse effects on some aspects of female reproduction ability which tested in female rodent. However, more evidence is needed to strengthen the conclusion.
With development of the industrial society, the population is more and more exposed to different kinds of chemical compounds. Some of them are known as endocrine disrupting chemicals (EDCs), which negatively affect human and environmental well-being [1, 2]. Phthalates esters, as one of the EDCs, are commonly used in polyvinyl chloride (PVC) based plastics production as plasticizers [3, 4]. Diethylhexyl phthalate (DEHP) is the most common phthalates ester, and the annual production level has reached to millions of pounds, which has the greatest endocrine disrupting potency [5, 6]. DEHP is easily transferred into the environment and bio-accumulated in the human body through ingestion, inhalation, dermal contacts, even intrauterine exposure [4, 5, 7, 8, 9, 10]. In the past few decades, many studies have proved that DEHP has negative effects on the male reproductive functions, such as variations of anogenital distance [11] and testicular toxicity [12, 13].
EDCs can also negatively affect the female reproductive functions such as trophoblast and placental function, the female hypothalamus-pituitary–gonadal axis, puberty onset and ovarian functions [14, 15]. The maternal exposure to EDCs even has some transgenerational effects [16]. DEHP, mainly through its secondary metabolites-mono(2-ethylhexyl) phthalate (MEHP), significantly affects the steroidogenesis and folliculogenesis through PI3K signaling pathway, 17b-hydroxysteroid dehydrogenase signalling pathway, peroxisome proliferator-activated receptors and changes the mRNA levels of cell cycle related proteins [3, 17, 18, 19, 20]. According to Fu et al. [21], the reproductive toxicity of DEHP/MEHP may be caused by the gut bacterial dysbiosis and altered metabolites, such as the alterations of short-chain fatty acid (SCFA), which is considered to be associated with the steroidogenesis dysfunction in patient with polycystic ovary syndrome (PCOS) [22]. However, little has been systematically investigated concerning the effects of DEHP/MEHP on folliculogenesis and ovarian steroidogenesis.
Based on the available studies with rodents, we conducted a systematic review with meta-analysis on the effects of DEHP/MEHP exposure on the folliculogenesis and ovarian steroidogenesis in the female rodents.
The methodology of this systematic review was pre-specified in a protocol, which has been published on the PROSPERO website in December, 2021 and the registration number is PROSPERO CRD42021292264. The applied methodology followed the guidelines of the Systematic Review Centre for Laboratory Animal Experimentation (SYRCLE) [23].
A comprehensive search strategy was used to identify articles in the EMBASE, PubMed, Web of Science, and Cochrance Library databases in December, 2021. The search strategy was provided in the supplementary material (Supplementary Table A.1). In addition, the reference lists of the included articles were screened manually in order to identify the potentially relevant new articles.
There were two screening phases in the study selection, namely initial screening based on the title and abstract, which was then followed by full-text screening of the eligible articles for final inclusion. In each phase, two observers (WH and YW) independently assessed each article. Discrepancies were resolved through discussion, or by consulting a third investigator (FQ).
The studies were included for full text screening when they met all the inclusion criteria: (1) original full paper presented unique data; (2) all female rodents (with all species and all ages) were exposed to DEHP/MEHP; (3) at least one of the following outcome measures related to folliculogenesis and ovarian steroidogenesis was examined (including ovary weight, serum estradiol levels, serum progesterone levels, mean estrous cyclicity, postnatal day (PND) of puberty onset, age of vaginal opening (VO)); (4) controlled studies with a separate control group. In case of doubt, a full text analysis was performed. During the full text screening, the studies were excluded if they met one of the following criteria: (1) duplicates; (2) not primary study; (3) the intervention/exposure of DEHP/MEHP not one of gavage, oral or inhalation; (4) without outcomes of interest (folliculogenesis and ovarian steroidogenesis related outcomes); (5) not a rodent study; (6) unhealthy rodents like animal model of disease.
Two review observers (WH and YW) independently extracted the bibliographic data from the included studies, including the characteristics of animal model, the exposure, the study design and the relevant outcome measures (i.e., ovary weight, serum estradiol levels, serum progesterone levels, follicle counting, oocyte counting, mean estrous cyclicity, PND of puberty onset, age of VO). If disagreement occurred, two authors discussed and arrived at a consensus with the investigator (FQ). We considered each analysis of a specific outcome measure with a specific dose as a separate comparison, however, when a study was carried two or more experiments in the same condition, we would merge the data with formulae. In addition, analyses in different ovaries (even derived from the same animal) and analyses with different time windows of exposure were considered as separate comparisons. Therefore, multiple comparisons from one study were included.
If the outcomes were repeatedly measured at different timepoint(s) in the same animals, we selected the timepoint at which the measured effect was greatest (i.e., the timepoint with the strongest association with the outcome). The timepoint of greatest efficacy was selected when the absolute difference between the mean of the exposure group and the mean of the control group divided by the sum of standard deviations (SDs) was highest. The direction of the effect was not considered when selecting the timepoint of greatest efficacy by using the absolute difference [24].
All data was collected as mean and SD. Standard error of the mean was recalculated to SD. In case the number of animals was unclear, a conservative estimate would be made, usually the mean. When the data were reported as median and interquartile range, the authors have contacted for raw data. The studies were excluded from the analyses when there existed missing data, unavailable author contact details, or no response from authors or remainders within 6 weeks.
The risk of bias of the included studies were assessed independently by two observers (WH and YW) using the SYRCLE’s Risk of Bias (RoB) tool (version 2, SYRCLE, http://WWW.SYRCLE.NL), which is specifically designed for animal studies [23]. Briefly, the ten items in the RoB tool were scored with “yes” indicating low risk of bias, “no” indicating high risk of bias or “? / unclear” indicating that the item was not reported and therefore the risk of bias was unknown.
Any discrepancies were resolved among the authors to reach a consensus. If, upon further discussion, the evaluation team couldn’t reach an agreement on a risk of bias determination for a particular domain, the more conservative judgment would be selected (i.e., if one observer made a judgment of “yes” and the other made a judgment of “? / unclear”, the “? / unclear” judgment would be used). The figure of risk of bias was performed using R (version 4.1.2, R Core Team, Vienna, Austria).
Meta-analyses were performed using Review Manager 5.4.1 (The Cochrane
Collaboration) [25]. For subgroup analysis, a minimum of 2 studies per subgroup
was required. If meta-analysis was not possible, data were reported through a
descriptive summary. We extracted the means and the SDs from each study. We
combined the means and the SDs when the difference of experiments in the study
were negligible. The following formula was applied to compute the pooled Mean:
Heterogeneity was assessed using I-square (I
The assessed subgroup variables included: animal species (rats or mice), strains, route of exposure (gavage, oral, diet, inhalation), dosage of treatment (below the tolerable daily intake (TDI) of 50 ug/kg/d [26], between 50 ug/kg/d and 5 mg/kg/d, between 5 mg/kg/d and 500 mg/kg/d, above 500 mg/kg/day, time window of exposure (perinatal exposure: during gestation and lactation period; prenatal exposure: during gestation only; postnatal exposure: during lactation period only), time of outcome measurement (before or after PND21, the normal weaning period), offspring of measure (F0: female mice before mated; F1: mice born form the F0 generation; F2: the F1 generation was mated with nontreated males to generate the F2 generation; F3: the F2 generation was mated with nontreated males to generate the F3 generation).
A sensitivity analysis method was conducted to evaluate the results. It is the latest timepoint was selected instead of the greatest timepoint [24].
The quality of the included studies was rated according to the confidence rating methodology of the Office of Health Assessment and Translation (OHAT) [27]. The available studies on a particular outcome were firstly grouped by the key study design features then each grouping of the studies was given an initial confidence rating according to those features [28]. According to the OHAT, an initial high confidence could be downgraded to a moderate, low or very low confidence based on assessment of five factors (i.e., risk of bias, unexplained inconsistency, indirectness, imprecision and publication bias). In addition, four factors could help to increase confidence in the results. As the factor “plausible confounding” primarily applied to the observational studies, the factor was not accessed [28]. Furthermore, the factor “large magnitude of effect” was also not assessed. According to the Wassenaar et al. [24], it was difficult to define “large effect”, as a relatively low dose of exposure led to major public health problems. Funnel plots were used to examine the publication bias when at least 5 studies could be included.
A flow chart of the study was provided in Fig. 1. Using the comprehensive electronic database search strategy, a total of 981 unique articles were identified from EMBASE, PubMed, Web of Science, and Cochrance Library databases. Finally, 15 articles were included in this systematic review after screening, of which, 8 reported the effects on ovary weight [29, 30, 31, 32, 33, 34, 35, 36], 6 on the serum estradiol levels [19, 29, 32, 37, 38, 39], 5 on the serum progesterone levels [19, 29, 32, 37, 38], 3 on the mean estrous cyclicity [29, 30, 32] and age of VO [39, 40, 41], 2 on the oocyte counting [39, 42] and PND at puberty onset [39, 40]. The detailed characteristics of all the included studies were provided in Supplementary Table A.2.
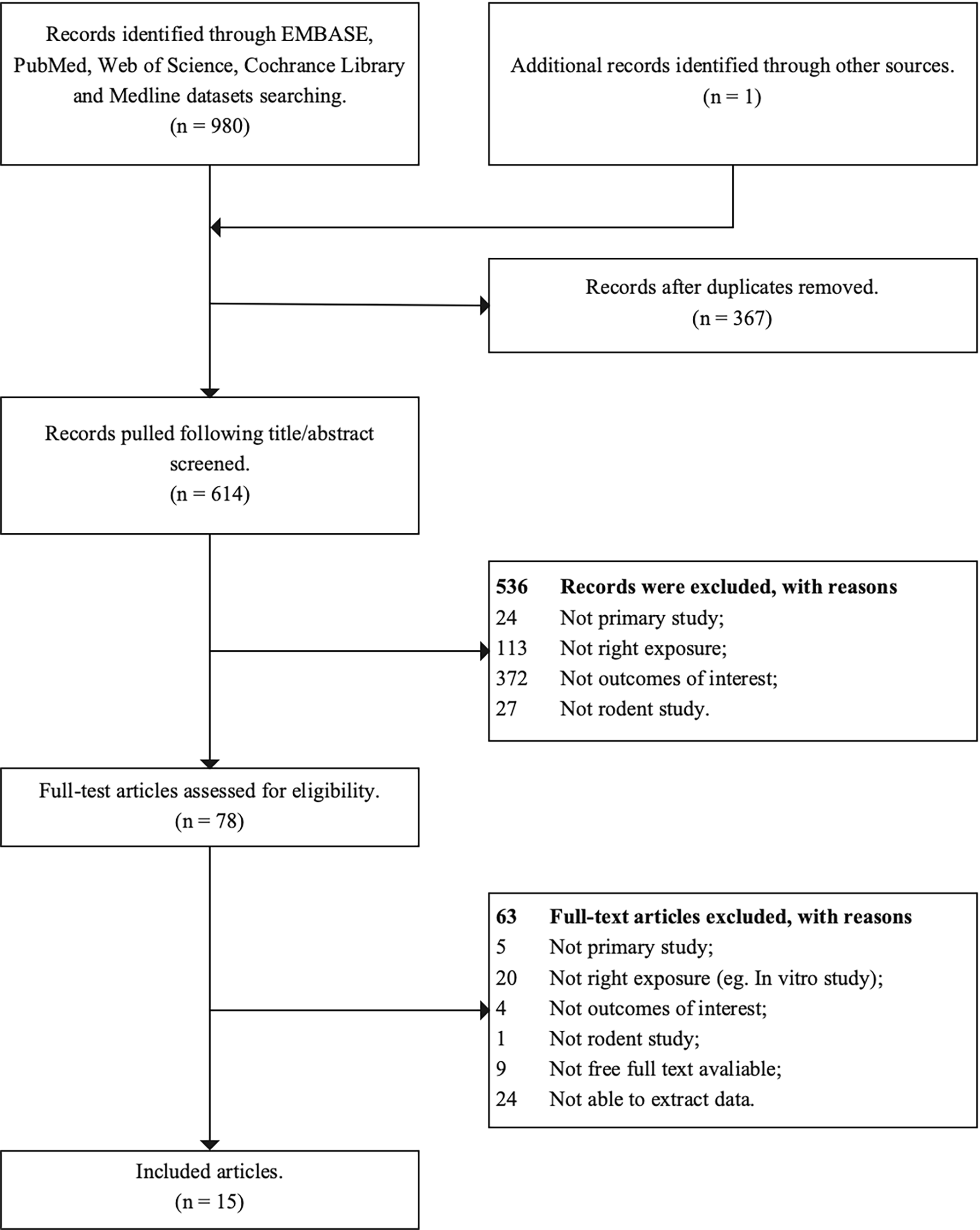
The flow chart of study selection process.
Using the SYRCLE’s RoB tool (version 2, http://WWW.SYRCLE.NL), the main observation from the risk of bias was the existence of many “? / unclear” scores, which means that most items were not sufficiently reported, resulting in an unknown risk of bias. The individual scores of the RoB tool of the included studies were provided in Fig. 2 and Table 1 (Ref. [19, 29, 30, 31, 32, 33, 34, 35, 36, 37, 38, 39, 40, 41, 42]). Compared to other bias, the selection bias was lower: the baseline characteristics and allocation concealment had 73% and 53% of low risk of bias respectively. The items more frequently recorded included random outcomes assessment (seven studies had a low risk of bias and one study had a high risk of bias), and incomplete outcome data (four studies had a low risk of bias and two had a high risk of bias).
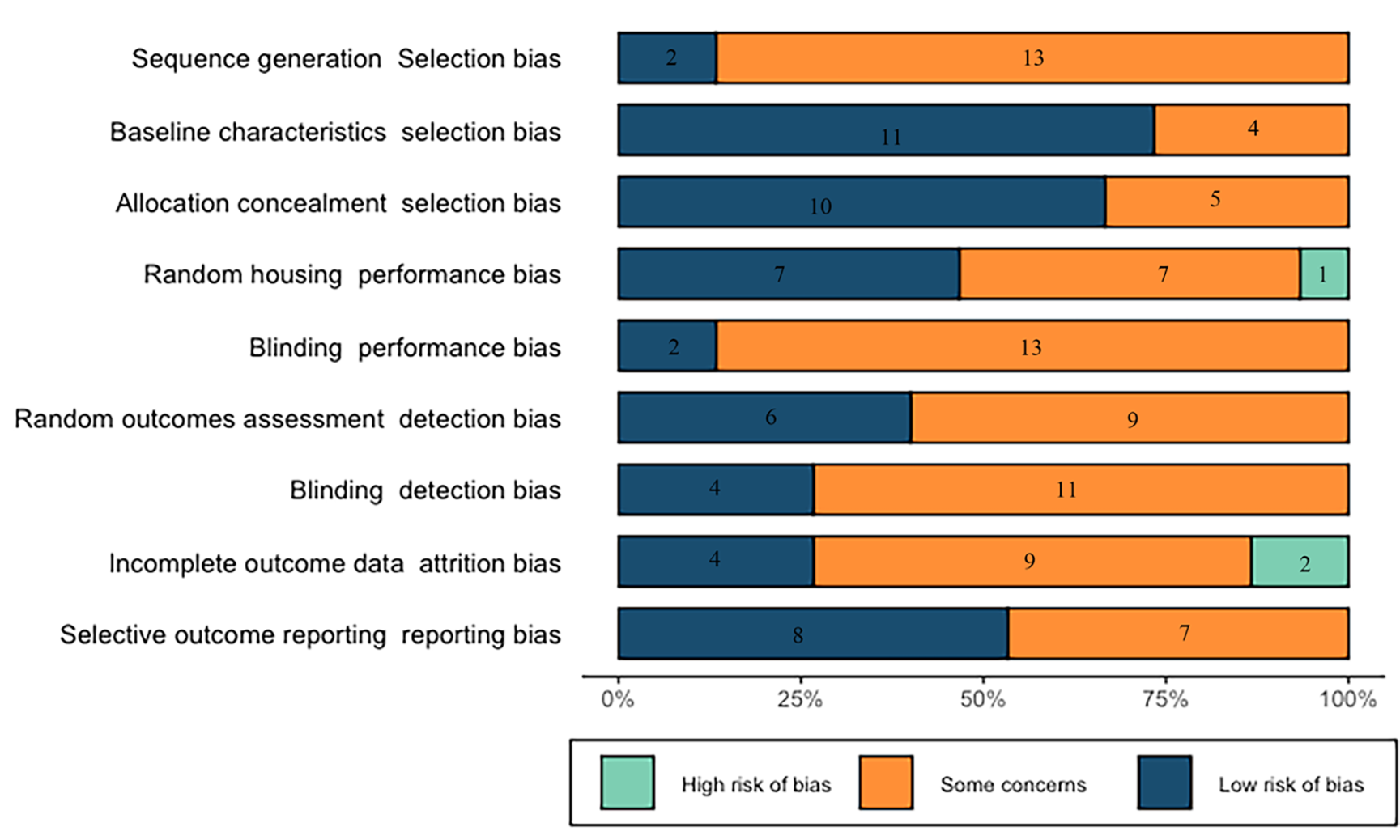
Results of the risk of bias and methodological quality indicators for all included studies.
Article info | Selection bias | Performance bias | Detection bias | Attrition bias | Reporting bias | Other | ||||
Sequence generation (selection bias) | Baseline characteristics (selection bias) | Allocation concealment (selection bias) | Random housing (performance bias) | Blinding (performance bias) | Random outcomes assessment (detection bias) | Blinding (detection bias) | Incomplete outcome data (attrition bias) | Selective outcome reporting (reporting bias) | Other sources of bias (other) | |
Grande et al. 2007 [29] | ? | Y | Y | ? | ? | Y | ? | Y | ? | ? |
Berman et al. 1993 [37] | ? | Y | Y | Y | ? | Y | Y | ? | Y | N |
Takai et al. 2009 [30] | ? | Y | Y | N | ? | ? | ? | ? | ? | ? |
Li et al. 2012 [38] | ? | Y | Y | Y | Y | Y | Y | Y | Y | ? |
Ma et al. 2006 [40] | Y | Y | ? | ? | ? | ? | ? | Y | ? | ? |
Pocar et al. 2012 [31] | ? | Y | ? | Y | ? | ? | Y | ? | Y | Y |
Pocar et al. 2017 [42] | ? | Y | ? | Y | ? | ? | Y | ? | Y | Y |
Tassinari et al 2021 [41] | ? | Y | Y | Y | ? | Y | ? | ? | Y | ? |
Yu et al. 2020 [32] | Y | Y | Y | ? | ? | ? | ? | ? | ? | Y |
Brehm et al. 2018 [33] | ? | ? | Y | Y | ? | Y | ? | ? | Y | ? |
Rattan et al. 2018 [36] | ? | ? | Y | ? | Y | ? | ? | N | ? | ? |
Niermann et al. 2015 [34] | ? | ? | ? | Y | ? | ? | ? | N | Y | ? |
Moyer et al. 2012 [39] | ? | ? | ? | ? | ? | ? | ? | ? | ? | ? |
Li et al. 2020 [19] | ? | Y | Y | ? | ? | Y | ? | Y | Y | ? |
Svechnikova et al. 2007 [35] | ? | Y | Y | ? | ? | ? | ? | ? | ? | ? |
Notes: The items in the SYRCLE’s RoB were scored with “yes” indicating low risk of bias, “no” indicating high risk of bias or “? / unclear” indicating that the item was not reported and therefore the risk of bias was unknown. Y, Yes; N, No.
A total of 15 studies including 61 comparisons investigated the effects of
DEHP/MEHP exposure on the ovary weight in this meta-analysis and the forest plots
for subgroup analysis were showed in Supplementary Figs. A.1–8 in
supplementary material. Overall, the DEHP/MEHP exposure significantly increased
the ovary weight in female rodents (mean
difference (MD) = 0.01; 95% confidence
interval (CI): 0.00, 0.02; p = 0.003; Table 2), with a substantial
heterogeneity among the studies (I
Analysis | Subgroups | MD |
I |
# studies (# comparisons) | Test for subgroup differences |
Ovary weight (mg) | |||||
Overall | 0.01 [0.00, 0.02] | 97% | 8 (61) | p = 0.003 | |
Species | Rats | 0.06 [–0.07, 0.18] | 100% | 4 (19) | p |
Mice | –1.81 [–2.45, –1.18] | 96% | 4 (32) | ||
Strain | CD-1(mice) | –1.81 [–2.45, –1.18] | 96% | 4 (32) | p |
Sprague-Dawley (r) | 0.00 [0.00, 0.01] | 89% | 4 (19) | ||
Route of exposure | Gavage | 0.00 [0.00, 0.01] | 92% | 2 (12) | p |
Oral | –1.49 [–2.22, –0.76] | 95% | 5 (26) | ||
Dose of exposure | DEHP |
0.44 [0.29, 0.59] | 99% | 5 (10) | p |
0.05 mg/kg/day |
0.01 [–0.00, 0.02] | 95% | 6 (13) | ||
5 mg/kg/day |
0.00 [–0.00, 0.01] | 91% | 7 (17) | ||
DEHP |
–1.34 [–2.63, –0.05] | 59% | 4 (11) | ||
Time window of exposure | Prenatal | 0.01 [0.01, 0.02] | 99% | 2 (12) | p |
Postnatal | –2.31 [–3.13, –1.49] | 84% | 3 (30) | ||
Offspring of measure | F0 | –2.08 [–4.80, 0.64] | 54% | 2 (7) | p |
F1 | 0.00 [–0.00, 0.01] | 91% | 6 (28) | ||
F2 | –3.12 [–4.91, –1.32] | 68% | 2 (8) | ||
F3 | –4.70 [–7.34, –2.05] | 89% | 2 (8) | ||
Time of outcome measure (F1) | PND0-PND21 |
–0.10 [–0.87, 0.67] | 95% | 2 (10) | p = 0.76 |
0.01 [0.01, 0.02] | 97% | 6 (32) | |||
Serum estradiol levels (ng/mL) | |||||
Overall | –1.34 [–4.01, 1.33] | 91% | 6 (26) | p = 0.32 | |
Species | Rats | –1.68 [–4.84, 1.48] | 93% | 4 (20) | p = 0.61 |
Mice | –0.25 [–4.83, 4.34] | 76% | 2 (6) | ||
Strain | Wister (rats) | –2.71 [–4.35, –1.07] | 61% | 2 (13) | p = 0.36 |
Sprague-Dawley (r) | 2.30 [–6.55, 11.14] | 96% | 2 (7) | ||
Others |
–0.25 [–4.83, 4.34] | 76% | 2 (6) | ||
Route of exposure | Gavage | –0.21 [–2.89, 2.47] | 92% | 4 (20) | p = 0.41 |
Oral | –5.65 [–18.30, 7.00] | 77% | 2 (6) | ||
Dose of exposure | 0.05 mg/kg/day |
3.74 [–1.71, 9.19] | 92% | 2 (6) | p = 0.03 |
5 mg/kg/day |
–5.15 [–8.94, –1.37] | 85% | 4 (9) | ||
DEHP |
–1.97 [–8.98, 5.04] | 94% | 3 (8) | ||
Serum progesterone levels (pg/mL) | |||||
Overall | –1.90 [–3.00, –0.79] | 94% | 5 (26) | p = 0.0008 | |
Strain | Sprague-Dawley (r) | –0.17 [–1.53, 1.18] | 95% | 2 (7) | p = 0.005 |
Wister (rats) | –3.38 [–5.13, –1.63] | 93% | 2 (13) | ||
Dose of exposure | 0.05 mg/kg/day |
0.10 [–1.01, 1.21] | 83% | 2 (6) | p |
5 mg/kg/day |
–2.21 [–3.57, –0.85] | 85% | 4 (9) | ||
DEHP |
–11.40 [–16.25, –6.56] | 97% | 2 (6) | ||
Oocyte counting (oocyte/animal) |
|||||
Overall | 0.08 [–1.51, 1.67] | 56% | 2 (8) | p = 0.92 | |
Mean estrous cyclicity (No. of days) | |||||
Overall | 0.11 [–0.00, 0.22] | 74% | 3 (18) | p = 0.06 | |
Dose of exposure | 0.05 mg/kg/day |
0.10 [0.01, 0.19] | 0% | 2 (5) | p = 0.38 |
5 mg/kg/day |
0.23 [–0.04, 0.50] | 88% | 3 (7) | ||
PND of puberty onset (days) |
|||||
Overall | 0.59 [–2.16, 3.35] | 93% | 2 (5) | p = 0.67 | |
Age of VO (days) |
|||||
Overall | –0.78 [–1.39, –0.17] | 64% | 3 (8) | p = 0.01 |
Notes: Effect size are expressed in MD with 95% CI calculated using
random-effects model. From each study, we considered each analysis of different
time, dose, offspring as individual comparison. Heterogeneity is measured by
I
As for route of exposure, the exposure through gavage didn’t significantly
increase the ovary weight, while oral exposure significantly decreased the ovary
weight in female rodents, which mainly through pipette feeding (p
Prenatal exposure significantly increased the ovary weight (MD = 0.01; 95% CI =
0.01, 0.02), while postnatal exposure significantly decreased the ovary weight
(MD = –2.31; 95% CI = –2.63, –0.05; p
In total, 6 studies consisting of 26 comparisons reported the effects of
DEHP/MEHP exposure on the serum estradiol levels
and the forest plots for subgroup analysis
were showed in Supplementary Figs. B.1–5 in supplementary material.
Overall, the exposure of DEHP/MEHP didn’t significantly decrease the serum
estradiol levels (MD = –1.34; 95% CI = –4.01, 1.33; p = 0.32; Table 2). The subgroup analyses showed that the heterogeneities were very high
(i.e., I
The exposure of DEHP/MEHP didn’t significantly change the serum estradiol levels for subgroup analysis of the route of exposure (p = 0.41) and dose of exposure (p= 0.03). However, the exposure significantly decreased the serum estradiol levels for the dose between 5 mg/kg/day and 500 mg/kg/day (MD = –5.15; 95% CI = –8.94, –1.37) or the dose higher than 500 mg/kg/day (MD = –1.97; 95% CI = –8.98, 5.04).
A total of 26 comparisons from 5 studies, which reported the serum progesterone
levels, were included and the forest plots for subgroup analysis were showed in
Supplementary Figs. C.1–3 in supplementary material. Overall, the
exposure of DEHP/MEHP significantly decreased the serum progesterone levels (MD =
–1.90; 95% CI = –3.00, –0.79; p = 0.0008; Table 2). The subgroup
analyses showed that for all estimates, the heterogeneities were quite high
(i.e., I
For subgroup of dose of exposure (p-value for subgroup differences
Three studies consisting of 18 comparisons reported the mean estrus cyclicity and the forest plots for subgroup analysis were showed in Supplementary Figs. D.1–2 in supplementary material. Overall, DEHP/MEHP exposure didn’t significantly prolong the mean estrus cyclicity (MD = 0.11; 95% CI = –0.00, 0.22; p = 0.06; Table 2). In subgroup analysis of dose of exposure (p = 0.38), the exposure didn’t significantly prolong the mean estrus cyclicity for the dose between 0.05 mg/kg/day and 5 mg/kg/day (MD = 0.10; 95% CI = 0.01, 0.19; p = 0.02) or the dose between 5 mg/kg/day and 500 mg/kg/day (MD = 0.23; 95% CI = –0.04, 0.50; p = 0.09).
A total of two studies consisting of 8 comparisons examined the DEHP/MEHP exposure on the oocyte counting and the forest plot for subgroup analysis was showed in Supplementary Fig. E.1 in supplementary material. Overall, DEHP/MEHP exposure didn’t significantly increase the oocyte counting (MD = 0.08; 95% CI = –1.51, 1.67; p = 0.92; Table 2). After 14 hours culture of the oocyte from the maternally treated female offspring, about 10% fewer oocytes reached MII stage in the exposure groups when compared with the controls, and the percentage of degenerated oocytes was nearly doubled [31]. The negative effect of maternal exposure to 0.05 mg/kg/day DEHP on oocyte development could still be detected in F2, F3 [42].
Five comparisons from 2 studies focused on the effects of DEHP/MEHP exposure on the PND of puberty onset and the forest plot for subgroup analysis was showed in Supplementary Fig. F.1 in supplementary material. DEHP/MEHP exposure did not significantly postpone the PND of puberty onset (MD = 0.59; 95% CI = –2.16, 3.35; p = 0.67; Table 2). The DEHP inhalation postponed the age of first estrus [40] and exposure of MEHP induced a four-day delay in the puberty onset of F1 adult female rodents [39].
Three studies, consisting of 8 comparisons, reported the DEHP exposure on age of VO and the forest plot for subgroup analysis was showed in Supplementary Fig. G.1 in supplementary material. Overall, the exposure significantly delayed the age of VO (MD = –0.78; 95% CI = –1.39, –0.17; p = 0.01; Table 2). In both of the 500 mg/kg/day and 1000 mg/kg/day MEHP exposure groups, a trend of delay was observed [39]. As for DEHP exposure, both inhalation [40] and gavage [41], the age of VO were delayed.
The overall effect of the exposure on ovary weight was not changed with the sensitivity analyses using the data from the latest measured timepoint (MD = –0.01; 95% CI = 0.00, 0.02; Supplementary Table A.2). However, for subgroup analyses (i.e., species, strain, route of exposure, dose of exposure, time of exposure measure, time window of exposure, offspring of measure), the effect for ovary weight was changed from a significantly negative effect to a non-significantly negative effect, so we used the latest measured timepoint, instead of the greatest measured timepoint, for the subgroup analyses. Sensitivity analyses were not conducted for other measure results because they didn’t contain the latest measure time studies [34, 36].
An initial confidence rating for all the seven outcomes were “high confidence”
(Table 3, Ref. [27]), because nearly all the studies had four features: controlled exposure,
exposure prior to outcome, individual outcome data, and comparison group used.
However, the quality of evidence for all the outcome measures were downgraded
because there were many “unclear” scores for the risk of bias (Table 1). In
addition, the confidence of all the outcome measures was downgraded with respect
to unexplained inconsistency because of the substantial heterogeneity
(i.e., I
Outcome measures | Body of evidence (Animal studies) | Factors for downgrading | Factors for upgrading | Final confidence rating | |||||||
Risk of bias | Unexplained inconsistency | Indirectness | Imprecision | Publication bias | Magnitude |
Dose response | Residual confounding |
Consistency species | |||
Ovary weight (mg) | Initial high confidence (8 studies) | ↓ |
↓ |
- | – |
– |
- | - | - | - | Very low |
Serum estradiol levels (pg/mL) | Initial high confidence (6 studies) | ↓ |
↓ |
- | – |
↓ |
- | - | - | - | Very low |
Serum progesterone levels (ng/mL) | Initial high confidence (5 studies) | ↓ |
↓ |
- | – |
↓ |
- | - | - | - | Very low |
Mean estrous cyclicity (No. of days) | Initial high confidence (3 studies) | ↓ |
↓ |
- | – |
- | - | - | - | - | Low |
Oocyte counting (oocyte/animal) | Initial high confidence (2 studies) | ↓ |
↓ |
- | – |
- | - | - | - | - | Low |
PND |
Initial high confidence (3 studies) | ↓ |
↓ |
- | - | - | - | - | - | - | Low |
Age of VO |
Initial high confidence (3 studies) | ↓ |
↓ |
- | – |
- | - | - | - | - | Low |
Notes: “ -” = no concern, or not present; “
Furthermore, for ovary weight, the publication bias was judged as undetected, according to the funnel plot (Supplementary Fig. A.9). The confidence for the serum levels of estradiol and progesterone were downgraded for publication bias, based on the funnel plots (Supplementary Fig. B.6 and Supplementary Fig. C.4). Consequently, the quality of evidence of ovary weight, the serum levels of estradiol and progesterone were “very low”. For other four outcome measures, the quality of evidence were “low”, which means the true effect may be different from the apparent relationship.
Many studies have proved the DEHP had adverse effects on the male reproduction system, however, the association between DEHP/MEHP exposure with female reproduction has not been clearly explored, therefore, we carried out this systematic review and meta-analysis.
The results of this systematic review and meta-analysis showed that DEHP/MEHP exposure significantly increased the ovary weight (p = 0.003), decreased the serum progesterone levels (p = 0.0008) and delayed the age of VO (p = 0.01), while the exposure didn’t significantly decrease the serum estradiol levels (p = 0.32). Additionally, DEHP/MEHP exposure did not significantly increase the oocyte counting (p = 0.92), prolong the mean estrus cyclicity (p = 0.06) and delay the PND of puberty onset (p = 0.67).
DEHP was identified as an index compound, since it has the most robust underlying toxicological dataset, therefore, the current TDI of DEHP at 0.05 mg/kg bw per day was reaffirmed in 2019 by the EFSA Food Contact Materials, Enzymes and Processing Aids (CEP) Panel’s updated opinion [26]. The “dose of exposure” subgroup analysis for the ovary weight showed that the dose lower than 0.05 mg/kg/day significantly increased on ovary weight and the dose higher than 500 mg/kg/day significantly decreased the ovary weight. In addition, the exposure dose between 0.05 mg/kg/day and 5 mg/kg/day significantly prolonged the mean estrus cyclicity, while the exposure dose between 5 mg/kg/day and 500 mg/kg/day significantly decreased the serum levels of estradiol and progesterone. Additional data is necessary to enhance the credibility of the results and whether the current TDI is safe enough.
Other subgroup analyses for the ovary weight showed that the exposure of DEHP/MEHP significantly decreased the ovary weight in F2 and F3, indicating that the exposure has transgenerational effects [33, 36]. However, the subgroup analysis of offspring could not be carried out for other outcome measures because of the insufficient number of studies (Table 2). According to E. Brehm et al. [33] and S. Rattan et al. [36], this transgenerational effects also impacted the follicle numbers, estrus cyclicity, and ovarian steroidogenesis in the offspring.
However, due to the limitations of the available data and low evidence of quality, the results of subgroup analyses should be interpreted with caution.
This systematic review applied SYRCLE approach as the main methodology, which is specifically designed for animal studies. In addition, some formulas were used to calculate the data from the repeated experiments in the same study. Furthermore, we analyzed the transgenerational effects of DEHP/MEHP exposure.
The main limitation of this systematic review is that the amount of the included
studies is relatively small and the quality of the available data is low,
therefore, other outcome measure could not be included in this study. In
addition, there was significant heterogeneity across the included studies
(i.e., I
According to the funnel plots of the serum levels of estradiol and progesterone (Supplementary Fig. B.6 and Supplementary Fig. C.4), a strongly suspected publication bias was observed. The reasons for the potential publication bias may be partly attributed to the publication tendency for positive results. Meanwhile, the relatively strict inclusion criteria maybe also contribute to it.
This systematic review only focused on the rodents and excluded other species, although the rodents are specifically relevant for risk assessment purposes. Other species [43], even human-cell studies [44], would also be valuable addition to the conclusion. Out of the included studies, only the route of exposure of one study [40] is inhalation. Other routes, such as subcutaneous injection [45] and intraperitoneal injection [46] should also be fully explored.
The reason why the follicle counting and other outcome measures of folliculogenesis were not be included lied in the uncertainties, such as the time taken from each experiment, the freezing method, the slice angle, the person observed, and the data without uniform units. We excluded the mixture exposure of DEHP/MEHP and other reproduction toxicants (i.e. dibutyl phthalate, diisobutyl phthalate, butyl benzyl phthalate), because the composition and dosage of substances in different experimental groups are different [47, 48].
The DEHP/MEHP exposure has adverse effects on some aspects of female reproduction ability which tested in female rodent. However, more evidence is needed to strengthen the conclusion.
WH—extraction of the data, drafting and revising the manuscript; WH, YW, YZ and FW—analysis of data; FQ—designing of the study, drafting and revising the manuscript. All authors contributed to editorial changes in the manuscript. All authors have participated sufficiently in the work and agreed to be accountable for all aspects of the work. All authors read and approved the final manuscript.
Not applicable.
Not applicable.
This study was supported by the Zhejiang Province Science Foundation for Key Program (LZ21H270001).
The authors declare no other conflict of interests. Fan Qu is serving as one of the Guest editors of this journal. We declare that Fan Qu had no involvement in the peer review of this article and has no access to information regarding its peer review. Full responsibility for the editorial process for this article was delegated to Antonio Ragusa, Alessandro Svelato and Caterina De Luca.
Publisher’s Note: IMR Press stays neutral with regard to jurisdictional claims in published maps and institutional affiliations.