- Academic Editor
Background: Caspase-3 is involved in the execution of apoptosis and is
widely used as an apoptotic marker. Tumor necrosis factor-
Preterm premature rupture of membranes (PPROM) is defined as the fetal membrane rupture before labor and 37 weeks of gestation. This complication is the identifiable leading cause of preterm birth and has been one of the main causes of infant morbidity and mortality [1, 2, 3]. Recently, term or preterm rupture of fetal membranes occurs not only as a result of the tensile and shear forces of uterine contractions, but also as the result of a weakening process of membranes. It has been reported that acute inflammation, in which proteolytic enzymes and activating cytokines are released, is effective in the subgroup of preterm infants with PPROM [4]. 26–50% of placentas born following PPROM have the characteristics of acute chorioamnionitis [5]. Romero et al. [6] reported that intraamniotic inflammation occurs in 37% of preterm birth cases before 37 weeks of gestation.
The healing mechanisms of damaged tissue are divided into four overlapping stages: (i) hemostasis, (ii) inflammation, (iii) migration and proliferation, and (iv) resolution and remodeling. Unlike adult tissues, fetal tissue is much simpler to heal [7]. Inflammation is minimized, fetal tissue is usually not vascularized, and granulation tissue is not formed. It provides rapid healing of fetal wound healing without leaving any trace [8]. Macrophages play an important role in the healing of fetal tissues. Circulating monocytes migrate to sites of injury where they differentiate into tissue macrophages, and tissue-resident macrophages are also involved in wound healing [9]. It has been reported that increased oxidative stress in women with premature rupture of membranes predisposes them to rupture by accelerating early cellular senescence, age-related inflammation, and proteolysis. One of the factors involved in the endogenous and exogenous mechanisms associated with the increased risk of PPROM is the occurrence of programmed cell death or apoptosis [10, 11].
Caspases are proteases that degrade functional and structural proteins in the
cell, leading to programmed cell death. They are synthesized as zymogens and
converted to proteases. Caspase-3 is involved in the execution of apoptosis and
is widely used as an apoptotic marker [12]. Tumor necrosis factor-
There is limited study on the PPROM from a histopathological perspective.
Considering the risk factors of PPROM, this study aimed to investigate the
molecular level signaling and histological examination of apoptotic and
inflammation processes (caspase-3 and TNF-
Ethical approval was taken from Dicle University Medical School, Non-interventional Clinical Trials Ethical Committee (2020/68). In our study, 25 healthy women and 25 women with PPROM were included. All placentas were obtained by the Department of Obstetrics with the help of the physician. All patients were informed about the data and experimental protocol. For each patient, biochemical and clinical parameters were noted after their approval. All patients read and signed informed consent form.
A total of 50 patients aged 20–40 years with a singleton pregnancy who were
admitted to the Department of Obstetrics were recruited, including 25 cases of
PPROM patients (gestational week
The diagnosis of PPROM was made using a speculum examination by visualizing the
amniotic fluid pooling in the posterior vaginal vault or amniotic fluid leakage
from the cervix, together with a positive test for the presence of Placental
Alpha Microglobulin-1 (Amnisure®) in vaginal fluid [14]. The
exclusion criteria of all pregnancies in the present study were: structural or
chromosomal abnormalities of the fetus, being at the active stage of labor,
previable rupture of membranes (
Placenta specimens were obtained from the chorion frondosum of the maternal surface of the placenta during the cesarean delivery. Fetal membrane specimens were acquired near the fetal membrane rupture. Placental samples were maintained in sterile conditions until the experiment, and deidentified by a physician. Samples were directly soaked into 10% formaldehyde solution and dissected for histological dye experiments. The placental tissues were later dehydrated in increasing alcohol series, soaked in xylol solution, and embedded in paraffin blocks. 4 µm sections were cut and stained for hematoxylin-eosin staining [15].
Placental sections were cleared in xylol solution, dehydrated in alcohol, and
cleared in distilled water. Epitope retrieval was inducted by ethylenediamine
tetra-acetic acid (EDTA) solution (pH = 8.0) for 15 minutes in a microwave oven
at 90 °C. After the sections were cooled down, they were rinsed in
phosphate-buffered saline (PBS) three times for 5 minutes. 3% hydrogen peroxide
(H
Statistical analysis was done using the IBM SPSS 25.0 software (IBM, Armonk, New
York, USA). The data were recorded as mean
Each section was analyzed with Image J software (version 1.53, LOCI, University
of Wisconsin, Madison, WI, USA). The staining intensity (expression) of caspase-3
and TNF-
We analyzed the demographic variables, clinical characteristics, and pregnancy outcomes between PPROM and control groups in Table 1. We found no significant differences between the groups regarding maternal age, gravidity, parity, body mass index, and the mean value of laboratory parameters, including hemoglobin, white blood cell, and C-reactive protein.
PPROM group (n = 25) | Control group (n = 25) | p-value | |
Maternal age, years | 28 (21–38) | 29 (22–39) | 0.613 |
Gravidity | 3 (1–6) | 3 (1–5) | 0.514 |
Parity | 2 (0–5) | 2 (0–4) | 0.143 |
Body mass index, kg/m |
27.5 (19.4–32.7) | 27.1 (19.8 |
0.877 |
Hemoglobin, g/dL | 10.88 |
11.26 |
0.209 |
White blood cell, /mm |
13.57 |
12.59 |
0.760 |
C-reactive protein, mg/L | 8.3 (0.9–61.2) | 7.14 (0.8–36.2) | 0.419 |
Latency period, days | 16.46 |
N/A | N/A |
Birth week | 31.88 |
38.36 |
|
Birth weight, g | 1982.6 |
3268.47 |
PPROM, Preterm premature rupture of membranes; N/A, Not available.
Histopathological and immunohistochemical staining of control and PPROM placentas were shown in Fig. 1. In the control group (Fig. 1a), the epithelium was slightly cuboidal at the location of the amniotic membrane and slightly protruding towards the lumen. The basement membrane was regular, the capillaries in the lower part were smooth and the endothelial cells were flat. In some areas in the lower part of the chorionic membrane, vacuolar structures, solitary dispersed inflammatory cell infiltrates, and prominent macrophage cells were seen. Few fibrinoid accumulations were observed. Syncytial nodes and bridges and blood vessels were observed to be regular (Fig. 1a). Inflammatory cell infiltrates were seen at the border of the chorioamniotic membrane in the PPROM group (Fig. 1b). Thinning of the vessel wall, thinning, and ruptures of the amniotic membrane, which is one of the typical features of premature rupture of membranes, were observed. Enlargement of the syncytial nodes, dilatation of the thinned vessels, congestion, and thrombosis were observed. It was observed that fibrinoid accumulation increased and vacuolar structures were prominent. Tears and increased cell infiltration were observed around the vessel (Fig. 1b).
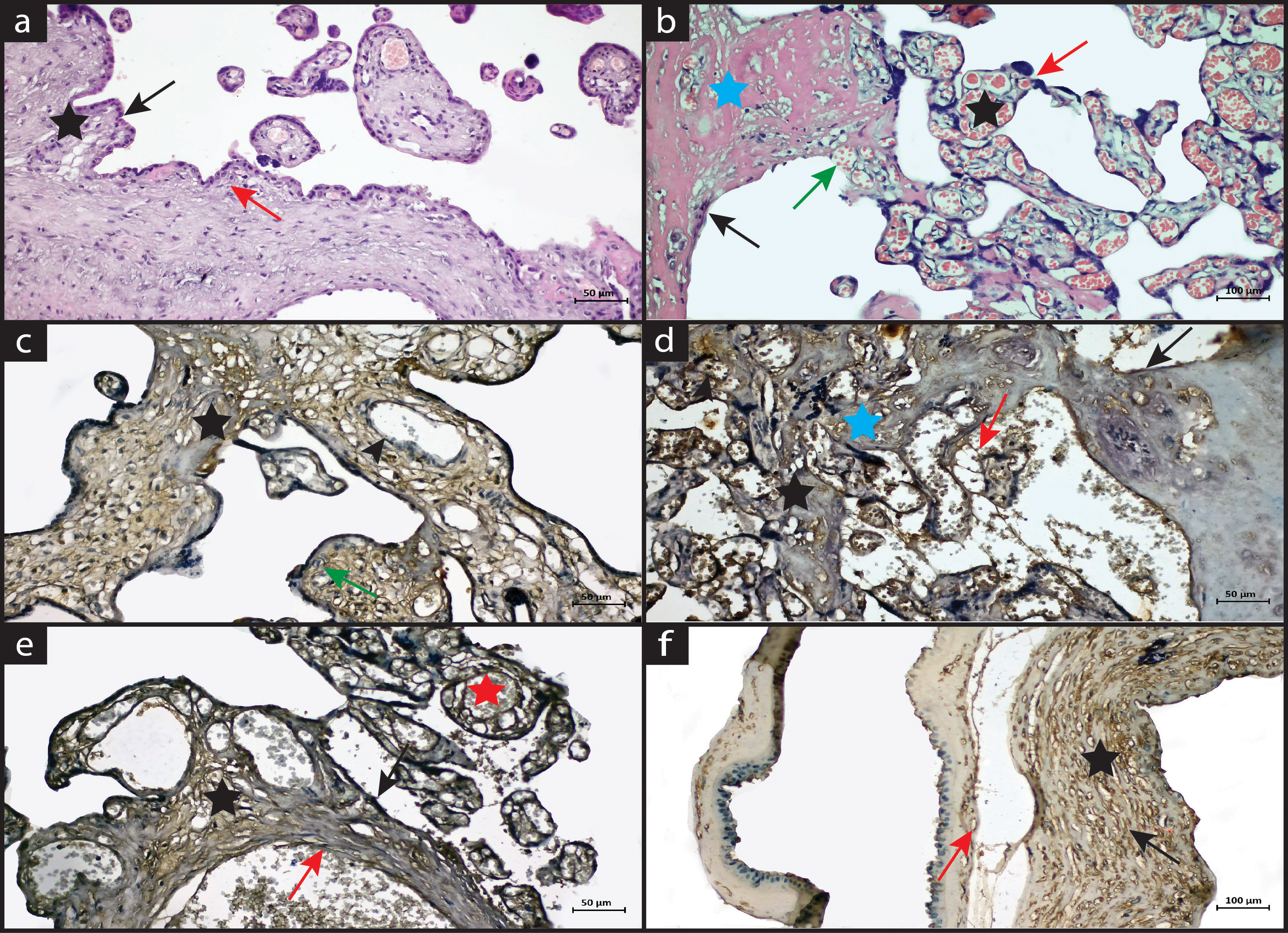
Hematoxylin eosin staining (a,b) and immunostaining (c–f) of the placental
sections. Bar: 50 µm, magnification: 20
In the control group, it was observed that the amniotic epithelium was regular, and caspase-3 expression was negative. Negative expression was also observed in syncytial nodes, vascular endothelium, and fibrinoid accumulation. Caspase-3 expression was also negative in Hoffbauer cells, especially in some areas where macrophage activity was evident (Fig. 1c). In the PPROM group, when the chorioamniotic membrane was examined, a caspase-3 positive reaction was observed especially in the interrupted amniotic membrane and in the dysfunctional amniotic epithelium. Thinning was observed in adjacent areas of the chorioamniotic membrane. Caspase-3 positive reaction was observed in some endothelial cells. Positive caspase-3 expression was detected especially in thinned areas, around the vessel wall, in fibrinoid accumulation, and in areas of inflammatory cell infiltration. However, caspase-3 showed negative expression in areas with syncytial nodes and syncytial bridges (Fig. 1d).
In the control group, negative TNF-
Staining intensity was done by Image J® software version 1.53.
Hematoxylin and DAB chromogen staining intensity for c-3 and TNF-
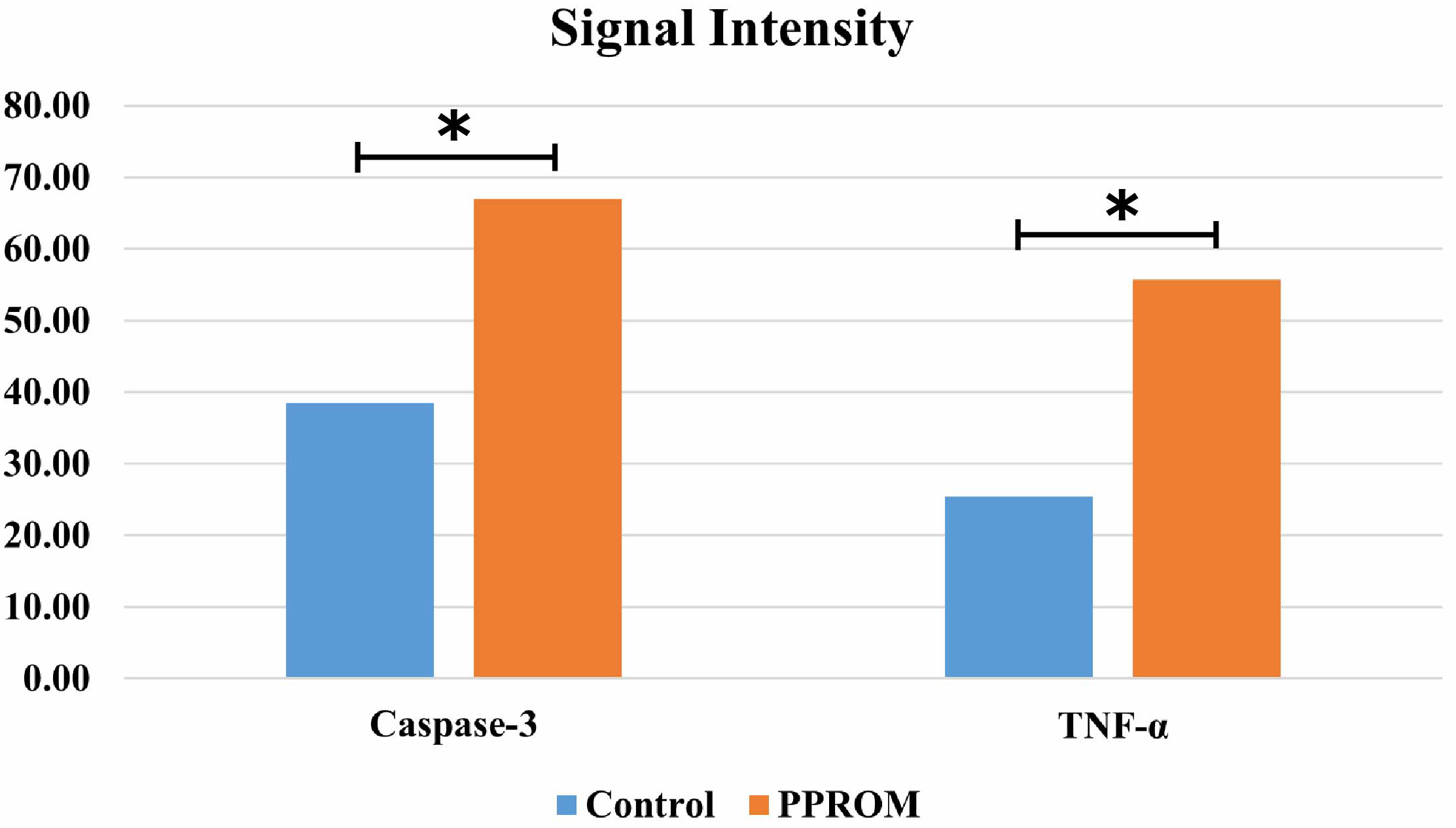
The immune activity of caspase-3 and TNF-
In this study, we found that the placentas of patients with PPROM showed
numerous pathologies such as loss of fetal membrane integrity, inflammation,
thinned vascular walls, and elevated fibrinoid accumulation. The caspase-3 and
TNF-
Of the fetal membranes, the inner and thinner one is the amnion, and the outer and thicker one is the chorion. Between the amnion and the chorion, there is a connective tissue rich in collagen. Amnion has more tensile strength than chorion. As pregnancy progresses, the weakening of the membranes occurs due to biochemical and biophysical changes. The amount of collagen decreases. The main mechanism in the rupture of membranes is the decrease in the amount of collagen in the structure of the membranes and the change in its composition [17, 18].
It has been reported that it may occur as a result of different factors, including physiological rupture of fetal membranes, mechanical modifications, remodeling of extracellular matrix components, cell degenerations, and apoptosis of amniotic cells. Since the amnion has poor vascularization, it has been shown that it cannot be applied for blood agglutination and epithelial wound healing in coagulation of the skin, and for reclosing the damaged amnion [19, 20]. It has been observed that the weakening of the extracellular matrix in the fetal membrane due to collagen degradation makes pregnant women active for PPROM. This process is thought to be the result of membrane stretching, which directly leads to tissue degeneration, as well as biochemical remodeling, inflammation, and apoptosis [21, 22]. In our study, the control group showed normal placental histology. Amniotic epithelium, vascular structures, and fibrinoid accumulation were regularly observed. Compared to the control group, dense inflammatory cell infiltration, thinned vessel walls with dilatation and congestion, increased syncytial nodes, and fibrinoid accumulation were observed in the PPROM group.
Caspase-3 is the main member of the caspase family involved in apoptosis. Before apoptosis begins, caspase-3 is activated, and almost all apoptosis proceeds by this signaling pathway. Immunohistochemical examination of the amniotic membrane in PPROM cases shows caspase-3 expression in amniotic epithelial cells and chorionic cytotrophoblast cells, while its expression is limited in mesenchymal and reticular cells of the matrix. This indicates that apoptosis occurs in the amnionic and chorionic layers. As previously described by Negara et al. [23], caspase-3 expression, which indicates apoptosis, was 9.75 times higher in amniotic epithelial cells in PPROM cases. In Harirah et al. [24] study on the role of apoptosis in the amniotic membranes of patients who delivered at term and patients who underwent cesarean section (artificial rupture), showed that the apoptotic index was three times higher in the region of spontaneous rupture in the chorionic trophoblast, with higher expression of active caspase-3. In our study, the control group showed mainly negative caspase-3 expression in placental components such as syncytial nodes, vascular endothelium, fibrinoid accumulation, and macrophages. In the PPROM group, caspase-3 positive reaction was increased in the amniotic membrane and epithelium, endothelial cells, fibrinoid accumulation, and areas of inflammatory cell infiltration. Moreover, we also observed that caspase-3 expression was higher in the placentas of patients with PPROM than in that of the control group.
PPROM induces choriodecidual inflammation. Bacterial invasion from the
choriodecidual space stimulates the release of cytokines such as TNF-
Weakening of the extracellular matrix in the fetal membrane due to collagen degradation makes pregnant women active in PPROM. This process appears to be a result of biochemical remodeling, inflammation, and apoptosis as well as membrane stretching, which directly leads to tissue degeneration.
Herein, we investigated the histopathology of the amniotic membrane and the role
of inflammatory cytokine TNF-
Data is available on request from the authors.
ED, FA, SCO, and GEA designed the research study. ISE, FA, GEA, and ÖK performed the research and experimental protocols. FA and SCO analyzed the data and statistics. ISE, ED and FA wrote the manuscript. FA and SCO made the critical review of the manuscript. All authors contributed to editorial changes in the manuscript. All authors have participated sufficiently in the work and agreed to be accountable for all aspects of the work. All authors read and approved the final manuscript.
Ethical approval was taken from Dicle University Medical School, Non-interventional Clinical Trials Ethical Committee (2020/68). All patients read and signed informed consent form.
Not applicable.
This research received no external funding.
The authors declare no conflict of interest. Süleyman Cemil Oğlak is serving as one of the Guest editors of this journal. We declare that Süleyman Cemil Oğlak had no involvement in the peer review of this article and has no access to information regarding its peer review. Full responsibility for the editorial process for this article was delegated to Michael H. Dahan.
Publisher’s Note: IMR Press stays neutral with regard to jurisdictional claims in published maps and institutional affiliations.