- Academic Editor
†These authors contributed equally.
Background: Recently, the utilization of sugar as a cryoprotectant has
garnered significant attention. Sucrose and trehalose, as non-permeable
cryoprotectants, can effectively regulate the osmotic pressure inside and outside
cells while maintaining cell membrane stability during cryopreservation.
Furthermore, it has been observed that monosaccharides sugars, particularly
fructose, exhibit superior efficacy in preserving the quality of frozen
substances as compared to disaccharides and trisaccharides
sugars. Nevertheless, there is currently limited research assessing the
cryopreservation effects of sucrose, trehalose, and fructose. The objective of
this experiment is to identify the best cryoprotectant between sucrose,
trehalose, and fructose for intact rat ovaries. Methods:
Intact ovaries with blood vessels were obtained from 20 adult
Lewis female rats and divided accordingly into four groups: control
(non-vitrified), sucrose, fructose, and trehalose. The ovarian samples were
subjected to a stepped cryoprotectant exposure and subsequently follicular
histological analysis using light and electron microscopy.
Ovarian cell apoptosis was evaluated by BCL2-Associated X (BAX)
immunohistochemistry and Terminal Deoxynucleotidyl Transferase-Mediated
Deoxyuridine Triphosphate nick End-Labeling (TUNEL) analyses. Results:
The sucrose and the trehalose groups preserved the ovarian histological structure
better than the fructose group. Additionally, there was no statistical difference
in the total follicle number between the sucrose and trehalose groups, but the
percentage of apoptotic cells in the trehalose group was significantly lower than
that of the sucrose group (p
Tumor diagnosis and treatment are constantly improving, enabling cancer patients to live longer. However, high doses of chemotherapy and/or radiotherapy used during tumor treatment can damage the female ovarian function, resulting in ovarian failure, infertility, and other issues that subsequently affect the female’s mental and social well-being [1, 2, 3]. Therefore, current research focuses on effectively protecting and preserving the reproductive function of the ovary while simultaneously treating tumors. Whole ovary cryopreservation and transplantation (WOCP&TP) offer a promising option for preserving reproductive endocrine function in young cancer patients [4]. In theory, the transplantation of intact ovaries with vascular anastomosis can efficiently restore blood supply, thereby reducing ischemic injury through a shortened ischemic period. Additionally, whole ovary transplantation provides a greater follicle reserve than cortical ovary transplantation, which potentially extends the functional lifespan of the transplanted ovary [4]. Currently, WOCP&TP have reported positive results in restoring endocrine function and successful delivery in mice, rats, rabbits, sheep, and other animals [5, 6, 7, 8]. The research on frozen intact ovarian transplants is still in its early stages, and there have been no documented cases of frozen transplants conducted on humans or primates. The transplantation of intact frozen ovaries in large animals has resulted in only three reported cases of live births [8, 9, 10], while rodents have reported only two successful instances [6, 11]. Therefore, extensive research is required before intact ovarian cryotransplantation can be fully implemented in the clinical setting. The main technical challenges in its implementation lie in the distribution of the cryoprotective agent (CPA) and its toxicity.
The commonly used CPAs for vitrification cryopreservation of ovarian tissue include permeable agents such as glycerol, ethylene glycol (EG), polyvinylpyrrolidone (PVP), dimethyl sulfoxide (DMSO), and propylene glycol (PROH), as well as non-permeable agents such as sucrose, trehalose, and proteins [12]. The combination of non-permeable and osmotic cryoprotectants can effectively reduce CPA toxicity, stabilize cell membrane integrity, and enhance the overall cryoprotection [13]. Non-permeable agents like sugars are small molecules that form polymers to increase extracellular osmotic pressure, leading to cellular dehydration, which acts as a stabilizing mechanism [14]. Simultaneously, sugars can maintain membrane integrity under low water activity conditions and mitigate CPA toxicity by forming hydrogen bonds with membrane phospholipids [15]. Sucrose is the most commonly utilized non-permeable CPA for vitrification and cryopreservation of animal ovaries [12, 16, 17]. Petrenko et al. [16] demonstrated that sucrose could partially replace DMSO in human fetal liver hematopoietic stem cells and human mesenchymal stromal cells. Additionally, during the slow freezing process, sucrose exerted a protective effect by binding to the membrane interface and/or influencing the water structure [17]. Trehalose is a natural non-reducing disaccharide that exhibits excellent performance in cryopreservation. It has been widely employed in low-temperature freezing of germ cells and reproductive tissues, making it a focal point of CPA research in recent years. A combination of trehalose and DMSO has proven effective for cryopreserving various cell lines, including stem cells, primary hepatocytes, and HepG2 cells [18]. In addition, trehalose vitrification has been reported to enhance the morphological preservation of human primordial follicles as compared to sucrose controls [15]. However, Naing et al. [19] discovered that during the cryopreservation of goat sperm, monosaccharides, particularly fructose, demonstrated superior cryopreservation on semen quality as compared to disaccharides and trisaccharides. Additionally, supplementation of raffinose with fructose or glycerin effectively protected the motility and both membrane and DNA integrities of freeze-thawed mouse sperms as compared to raffinose alone [20].
Despite numerous studies reporting the advantageous effects of sugar as a CPA, to our knowledge, there is currently no comparative analysis of its effects on intact ovarian cryopreservation. Therefore, this present study aimed to investigate the impact of three different cryoprotectants (sucrose, fructose, and trehalose) on intact ovarian cryopreservation via perfusion in a rat model and evaluate their influence on histological changes and apoptosis to determine the most effective CPA for preserving intact ovaries.
Healthy adult female Lewis rats (n = 20, 8–10 weeks old, and weighing 180–200 g) were purchased from Shanghai Sipur Bikai Experimental Animal Co., LTD. and kept under standard conditions (room temperature 23–25 °C, relative humidity 40%–60%, good ventilation, light/night cycle of 12 hours, free diet, and drinking water) at the Animal Experimental Center of Fudan University. The animals were acclimatized for one week after entering the room, and vaginal smears were performed for 5–7 consecutive days to determine the estrous cycle. Rats with regular estrous cycles were selected as experimental animals. Animal euthanasia method: animals are placed in a euthanasia chamber where carbon dioxide is introduced. The experimental animal is then monitored to ensure that all vital signs have completely disappeared, which is determined through assessments such as toe reflex. After confirming the absence of respiration and cardiac activity, proceed with the removal of the deceased individual. The study was approved by the Ethics Review Committee of the Obstetrics and Gynecology Hospital of Fudan University (Grant No. 202209027Z). The use of animals in our experiments met ethical requirements. All activities related to this research project were performed following the relevant guidelines and regulations of the Obstetrics and Gynecology Hospital of Fudan University. The research protocol has been submitted to PreclinicalTrials.eu. database https://preclinicaltrials.eu/?login_to=dashboard%2Fadd-protocol.
(i) 0 M CPA: M2 culture medium (M7167, Sigma Inc., Marlborough, MA, USA) + 10% (v/v) heat-inactivated fetal calf serum (FCS) (SH30406.05, Sigma Inc., Marlborough, MA, USA).
(ii) 0.5 M CPA: M2 culture medium + 10% FCS + 0.5 mol/L dimethyl sulfoxide (DMSO) (D1435, Sigma Inc., Marlborough, MA, USA).
(iii) 1 M CPA: M2 culture medium + 10% FCS + 1.0 mol/L DMSO + 0.1 mol/L sucrose (V900116, Sigma Inc., Marlborough, MA, USA).
M2 culture medium + 10% FCS + 1.0 mol/L DMSO + 0.1 mol/L fructose (F0127, Sigma Inc., Marlborough, MA, USA).
M2 culture medium + 10% FCS + 1.0 mol/L DMSO + 0.1 mol/L trehalose (T5251, Sigma Inc., Marlborough, MA, USA).
(iv) 1.5 M CPA: M2 culture medium + 10% FCS + 1.5 mol/L DMSO + 0.1 mol/L sucrose.
M2 culture medium + 10% FCS + 1.5 mol/L DMSO + 0.1 mol/L fructose.
M2 culture medium + 10% FCS + 1.5 mol/L DMSO + 0.1 mol/L trehalose.
(v) 3 M CPA: M2 culture medium + 10% FCS + 3.0 mol/L DMSO + 0.2 mol/L sucrose.
M2 culture medium + 10% FCS + 3.0 mol/L DMSO + 0.2 mol/L fructose.
M2 culture medium + 10% FCS + 3.0 mol/L DMSO + 0.2 mol/L trehalose.
The intact rat ovaries (n = 20) were randomly grouped, with five ovaries in each group.
Trehalose group: the non-permeable protective agent in the cryoprotectant was trehalose.
Fructose group: the non-permeable protective agent in the cryoprotectant was fructose.
Sucrose group: the non-permeable protective agent in the cryoprotectant was sucrose.
Fresh/control group: direct formaldehyde or glutaraldehyde fixation without any treatment.
The rats were anesthetized with 1% sodium pentobarbital
solution (40 mg/kg) (P3761, Sigma Inc., Marlborough, MA, USA)
by intraperitoneal injection and fixed in the supine position. The intact rat
ovary with vascular pedicle was obtained by the method of Wang et al.
[21] under an operating microscope with an 8
The intact ovary was transferred to the perfusion device and was injected with a low flow rate at linear incremental precision using our previously modified perfusion device and protocol [6]. Container 1 of the perfusion device contained a solution of 3 M DMSO CPA, container 2 contained a solution of 0 M DMSO CPA, and container 3 contained a solution of 1.5 M DMSO CPA. The solution from container 1 was pumped into container 2 at a flow rate of 0.15 mL/min, while the solution from container 2 was pumped into container 3 at a flow rate of 0.35 mL/min. The entire perfusion process lasted for a total of 20 min. We also ensured that the concentration of CPA perfused into the intact ovary increased linearly from an initial concentration of 0 M to a final equilibrium concentration of 1.5 M within the first 15 min, which was then maintained for the remaining 5 min.
At the end of perfusion, the entire ovary was transferred into a cryotube and placed in the cryoscope for gradual ultra-low temperature freezing, following the methodology described in our previous publication [6]. The initial temperature was set at 0 ℃ and gradually decreased to –7 ℃ at a rate of 2 ℃/min. After maintaining this temperature for 10 min, the temperature was reduced to –40 ℃ at a rate of 0.3 ℃/min and subsequently to –120 ℃ at a rate of 10 ℃/min. The centrifuge tube containing the sample was then immersed in a liquid nitrogen tank for storage, with a minimum duration of one week before evaluation or transplantation.
To initiate thawing, the ovaries were left at room temperature for 30 s, followed by immersion in a 40 °C water bath for 5 min. The thawed ovaries were reconnected to the perfusion device, and CPA was injected using the same flow rate as previously employed, thereby reversing the concentration gradient. Finally, they were stored in ice before testing.
The ovarian tissue was fixed with 4% paraformaldehyde, followed by dehydration
using gradient alcohol. Subsequently, it was made transparent and embedded in
paraffin. The tissue blocks were then continuously sectioned into 4–5 µm
sections. These sections were stained with hematoxylin and eosin (HE) [22] and
observed under an optical microscope (Olympus DP73, Olympus, Tokyo, Japan) at
200
The primordial, primary, secondary, and antral follicles were counted at
200
Tissue specimens were fixed in pre-cooled 2.5% glutaraldehyde at 4 °C for 2 hours and rinsed three times with 0.1 mol/L phosphate buffered saline (PBS) for 15 min each time. The tissues were then fixed in 1% osmium tetroxide for 1 h and rinsed three times with 0.1 mol/L PBS for 15 min each time. The tissue samples were subsequently washed with ethanol (50%, 70%, and 90%) for 15 min at each ethanol concentration, 100% ethanol for 20 min, and 100% acetone for 20 min. The tissue was permeated with anhydrous acetone and embedding agent at 1:1 volume and shaken for 2 h, followed by permeation with a pure embedding agent and shaken for 2 hours. After embedment, the samples were polymerized in an oven and sectioned accordingly. The ultra-thin sections (70 nm) were stained with 4% uranyl acetate for 20 min and lead citrate for 5 min. The nucleus, cytoplasm, mitochondria, and other ultrastructural and intercellular connections were observed under transmission electron microscopy [22].
The tissue was fixed with 4% paraformaldehyde, followed by
dehydration using gradient alcohol. Subsequently, it was made transparent and
embedded in paraffin. The tissue blocks were then continuously sectioned into
4–5 µm sections. Immunohistochemistry analysis of BAX was performed for
every tenth section using the BAX immunohistochemical kit
(Abcam Inc., Cambridge, London, England). Sections were routinely dewaxed and
hydrated. Subsequently, antigen repair was performed under high pressure for 15
min in sodium citrate buffer solution and placed in H
The tissue was fixed with 4% paraformaldehyde, followed by dehydration using gradient alcohol. Subsequently, it was made transparent and embedded in paraffin. The tissue blocks were then continuously sectioned into 4–5 µm sections. TUNEL analysis was performed for every tenth section using the TUNEL kit (Cat#: 12156792910, Roche Diagnostics, Tokyo, Japan). Sections were routinely dewaxed and hydrated. Proteases were digested in these sections, and 50 µL of TUNEL reaction mix was added dropwise until the diaminobenzidine (DAB) color was visible. The sections were then restained with hematoxylin, sealed after dehydration, and made transparent. For each group, PBS substituted the TdT enzyme as the negative control. A brownish-yellow granular deposition of cell membrane and cytoplasm was considered a positive result. The staining results were visualized under a light microscope (Olympus DP73, Tokyo, Japan), and the images were randomly selected from 5 fields of each section. For quantitative analysis, the image analysis software ImagePro Plus Vision 6.0 (Media Cybernetics, Rockville, MD, USA) was used. The percentage of TUNEL-positive cells was calculated as the number of brown cells out of the total number of cells.
The data obtained from the experiments was expressed as mean
Ovarian tissue sections were visualized by light microscopy after HE staining (Fig. 1). The histological structure of follicles, medullary stroma, and blood vessels were normal in the fresh control ovaries (Fig. 1a). Most of the follicles were normal in the sucrose (Fig. 1b) and trehalose groups (Fig. 1c), with occasional histologically abnormal follicles. However, more abnormal follicles were observed in the fructose group (Fig. 1d), which included wrinkled oocytes, pyknotic oocyte nuclei, vacuoles in the follicles, disrupted basement membrane integrity, and disorganized surrounding granulosa cells that were detached from the basement membrane.
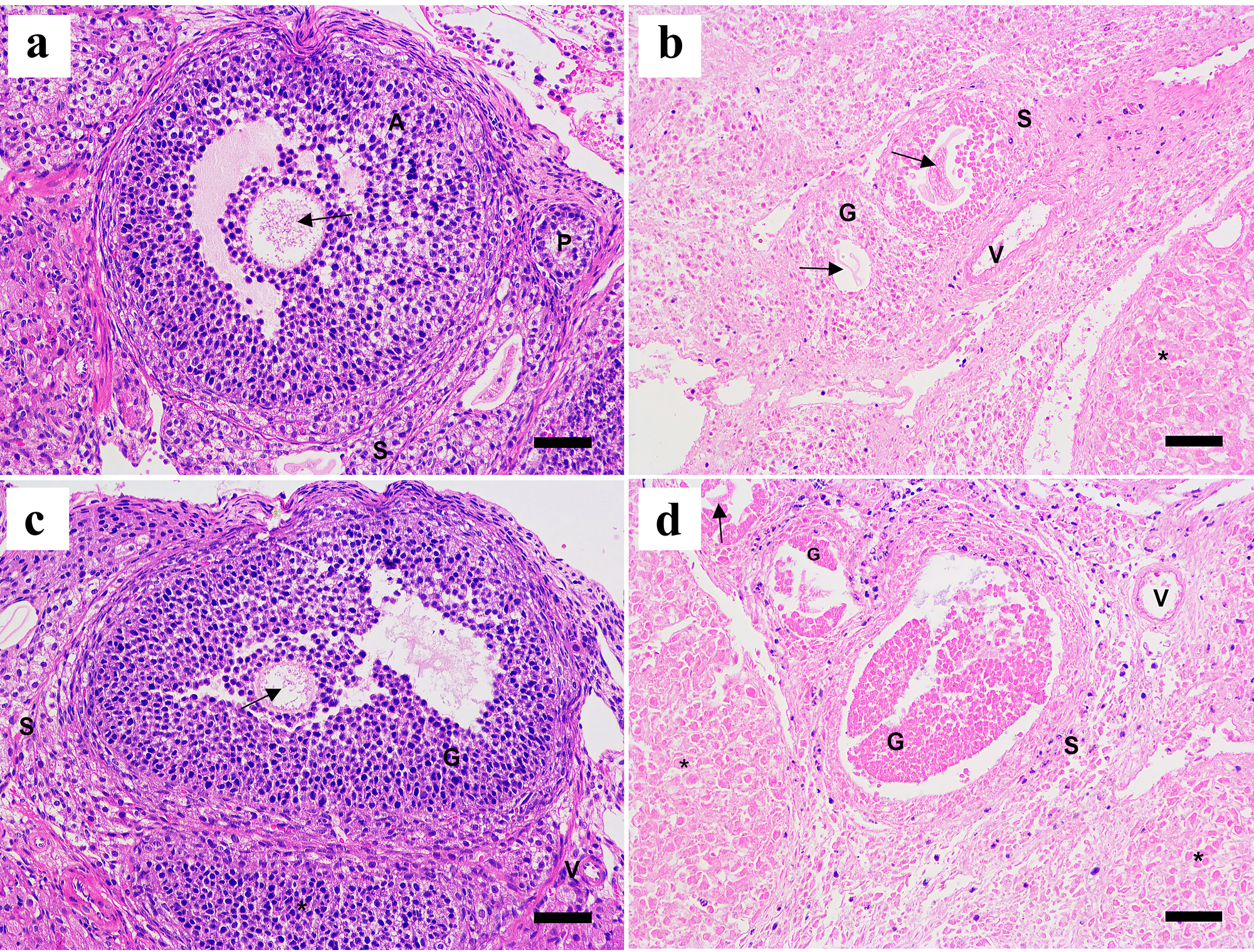
Representative histological images of
HE-stained ovaries of different groups at 200
The number of primordial and antral follicles in the sucrose group accounted for
77% and 74% relative to the number of follicles in the fresh group,
respectively (p
Group | The number of ovarian follicles of different types | Total number of follicles | |||
Primordial follicles | Primary follicles | Secondary follicles | Antral follicles | ||
Sucrose group (n = 5) | 79.6 |
22.8 |
9.8 |
5.0 |
118.2 |
Trehalose group (n = 5) | 81.2 |
22.0 |
8.8 |
5.0 |
119.6 |
Fructose group (n = 5) | 73.8 |
16.4 |
6.0 |
3.4 |
100.4 |
Control group (n = 5) | 104.6 |
27.6 |
12.4 |
6.8 |
151.4 |
Note: * p
Images (at 200
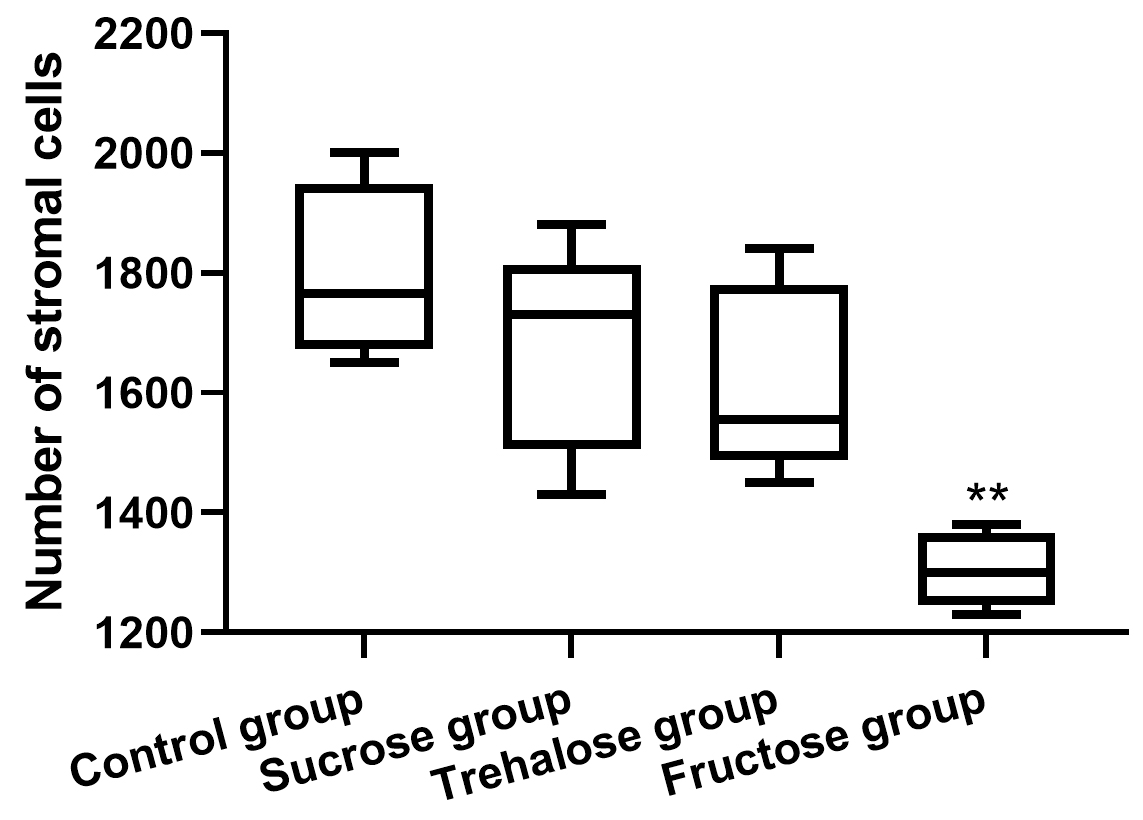
Quantitative analysis of stromal cells in each group (n = 5). Only
the fructose group was statistically different compared to the control
group (** p
The ultrastructure of the ovarian tissues of each group was observed under the electron microscope (Fig. 3). The nucleus and nucleolus of oocytes in the fresh group were visible. The boundaries of nuclei were clear and oval-shaped, and the chromatins were equally distributed in most areas of the nucleus. Many mitochondria were present in the cytoplasm (Fig. 3a). However, the mitochondrial cristae exhibited different degrees of damage, while the endoplasmic reticulum appeared normal with no obvious vacuoles. Both the sucrose (Fig. 3b) and trehalose groups (Fig. 3c) contained visible nuclei and nucleolus, but the nuclei in the trehalose group were of different sizes. Their nuclei were oval-shaped, and the chromatins were evenly distributed throughout the nucleus. Chromatin agglutination was occasionally observed at the edge of the nuclei. Likewise, the mitochondrial cristae were damaged to various degrees, and endoplasmic reticulum and vacuoles were observed in the cytoplasm. Furthermore, irregular nuclei were observed in the oocytes of the fructose group (Fig. 3d), and chromatin agglutination was also detected. Some mitochondria were swollen, and the mitochondrial cristae were damaged to varying degrees. Besides that, the cytoplasm contained more vacuoles than the other groups, and the granulosa cells also had pyknotic nuclei.
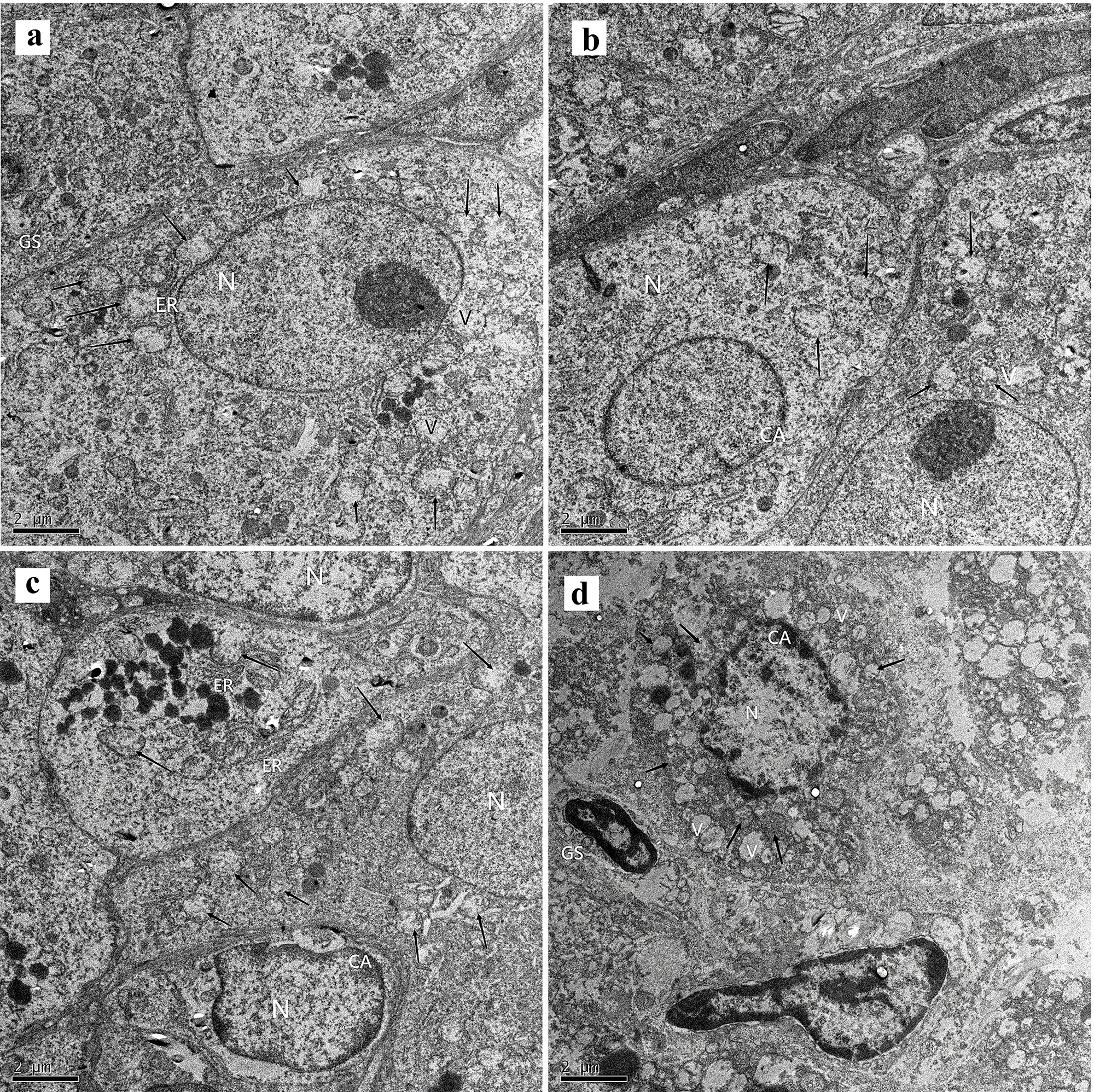
Ultrastructural changes in primordial follicles were observed in
each group (5000
BAX immunohistochemical and TUNEL analyses were performed on ovarian tissue
sections to assess the apoptosis of the follicles (Figs. 4,5). In the fresh
group, relevant positive signals (dark brown cells) were scarcely detected (Figs. 4a,5a). In the sucrose group, the TUNEL and BAX positive cells were mainly in the
granulosa cells of the antral follicles, as well as the secondary follicles and
corpus luteum (Figs. 4c,5c). The number of apoptosis cells of
the sucrose group was significantly higher than that of the control (p
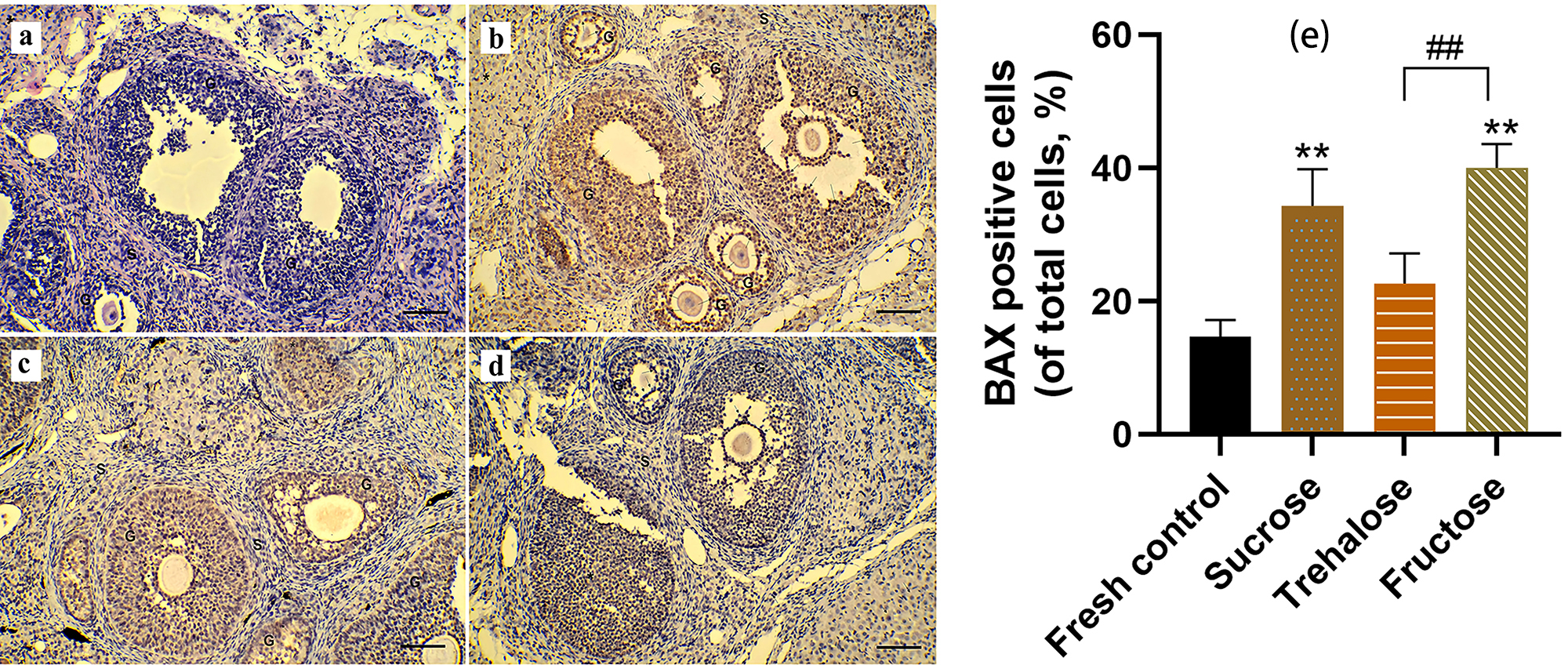
Cell apoptosis in ovarian tissue was assessed through BCL2-Associated X (BAX)
immunohistochemical analysis (200
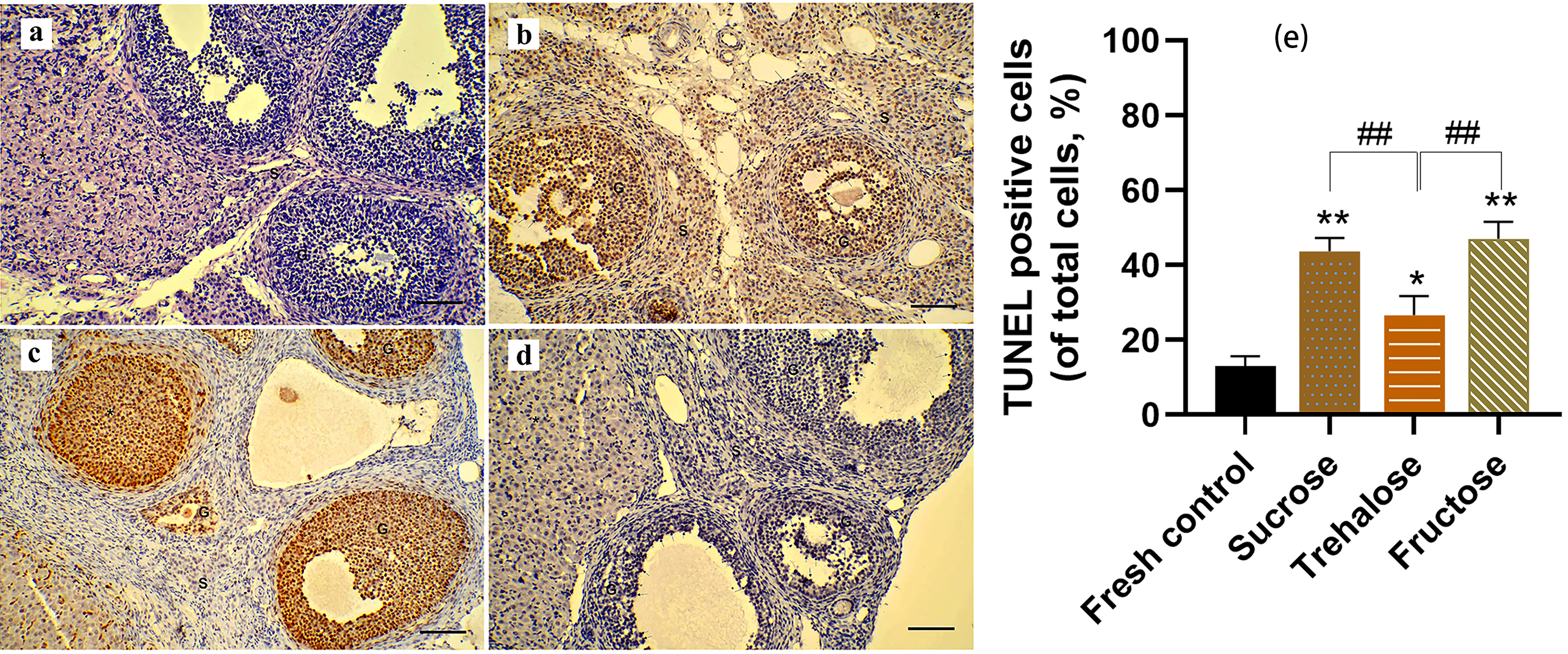
Cell apoptosis in ovarian tissue was assessed
through Triphosphate Nick End-Labeling (TUNEL) staining analysis (200
In this study, three different non-permeable cryoprotectants (i.e., sucrose, fructose, and trehalose) were compared to investigate their cryoprotective effect on the cryopreservation of intact rat ovaries. Our findings revealed that trehalose was the best cryoprotectant for the cryopreservation of intact rat ovaries.
Cryoprotection utilizes two types of CPAs: permeable and nonpermeable protectants [12]. The combination of these agents can effectively mitigate CPA toxicity, stabilize cell membrane integrity, and enhance low-temperature protection [13]. Currently, sucrose is the most commonly used impermeable protective agent. Fathi et al. [24] conducted a comparative study on seven different CPA mixtures based on DMSO, ethylene glycol, propylene glycol, and sucrose. Their findings concluded that the combination of sucrose, DMSO, and ethylene glycol exhibited significant advantages in terms of ovarian tissue structure preservation, follicle count, and initial follicle survival rate [24]. Likewise, trehalose has unique biological properties without imparting any toxicity to cells. It can enhance protein folding stability [25], form a distinctive protective film to stabilize cell membrane structure during dehydration on the cell surface [26], and protect cells and tissues through water substitution, glassy state, and chemical stability mechanisms [27]. Trehalose has found extensive applications in cryopreservation research involving germ cells [28, 29, 30, 31, 32] and tissues [33, 34, 35, 36], making it a prominent focus in CPA studies. Additionally, recent reports have indicated that fructose may confer a protective effect in the context of oocyte cryopreservation [37].
In this study, there were no significant differences observed in the integrity of the ovarian histological structure, follicular ultrastructure, follicular number, and stromal cell density between the sucrose and trehalose groups (total follicle count of 78.1% and 79% as compared to that of the fresh group, respectively), which was consistent with those reported by Tian et al. [15] who also found no significant difference in the protective effects of trehalose and sucrose during vitrification of ovarian tissue. However, the fructose group exhibited significant impairment in ovarian tissue structure and follicular ultrastructure, along with a notable decrease in the number of follicles and stromal cell density within antral follicles compared to the other groups. These findings indicate that fructose, among the three sugar-containing CPA, demonstrated a comparatively lower protective effect on intact frozen rat ovaries than sucrose and trehalose. Previous studies have demonstrated that perfusing ovaries with fructose and DMSO resulted in 80% endocrine function restoration [38] and 14% live birth rate [21] following the transplantation of thawed ovaries. It’s a pity that their studies failed to incorporate a comparative analysis of these effects with other sugars.
In previous studies, trehalose demonstrated potent anti-apoptotic properties, and its protective effect on freeze-thawed bovine ovarian granulosa cells could be attributed to its ability to enhance the expression of CYP2 and FSHR genes while inhibiting the expression of the BAX gene [39]. Mo et al. [40] discovered that trehalose mitigated heat stress (HS)-induced mitochondrial apoptosis in IPEC-J2 cells, leading to an elevation in mitochondrial membrane potential (MMP) and a reduction in the activity of pro-apoptotic proteins cysteine 3, Bax, and cytochrome c (Cyt c). Consequently, the TUNEL apoptosis test in this study revealed that the percentage of apoptotic cells was significantly lower in the trehalose group than in the sucrose group, which aligned with the Bax immunohistochemical findings. Furthermore, prior research has indicated that mitochondria preserved in trehalose buffers maintained both their integrity within the mitochondrial outer membrane (MOM) and their responsiveness towards bcl-2 family proteins. This preservation reduced their permeability and consequently inhibited the mitochondrial apoptotic pathway similar to that of fresh mitochondria [41]. These results suggested that trehalose may play diverse roles in suppressing the apoptotic signaling pathway, including the stabilization of ovarian cell membranes, preservation of mitochondrial structure and function, and downregulation of BAX expression. Therefore, trehalose would be a better alternative over sucrose for the cryopreservation of intact ovaries.
We used sucrose and trehalose at concentrations of 0.1–0.2 mmol/L in our study,
as higher concentrations of sucrose did not provide additional benefits, as
suggested in previous reports [6]. Tanpradit et al. [42] cultured the
fragments of the ovarian cortex in an L-15 medium supplemented with 1.5 M DMSO
and sucrose (0, 0.1, or 0.3 M). The sucrose slow-freezing group displayed a
higher number of viable ovarian follicles, while stromal cells reported better
viability at 0.1 M as compared to 0.3 M concentration. However, increasing the
sucrose concentration to 0.3 M did not yield better results than at 0.1 M
concentration [42]. The investigation on the slow freezing process of human
oocytes revealed that the utilization of 0.3 mmol/L sucrose resulted in
pronounced shrinkage of oocytes during dehydration stages, as compared to the
application of 0.1 mmol/L and 0.2 mmol/L sucrose solutions. This phenomenon may
potentially induce cellular damage and consequently diminish overall viability
[43]. Moreover, Marques et al. [36] found that the slow freezing 4 (SF4)
solution (comprising of 2 M methanol, 0.1 M trehalose, and 10% yolk solution)
displayed the highest percentage of membrane integrity (63.5%
In this study, we examined the histological structure and apoptosis of the ovaries and validated the cryoprotective effects of three different sugars at low temperatures. However, our article exhibits certain limitations, primarily due to the absence of a vitrification frozen group (without the infusion of any of the 3 sugars) as a positive control, thereby somewhat constraining the scope of our conclusions. We did not investigate the recovery of ovarian function, and we did not evaluate the precise mechanisms underlying the beneficial effects observed during perfusion in cryopreservation. Moreover, it should be noted that rat ovaries are considerably smaller than human ovaries and, thus, could better tolerate the experimental procedures employed in this study. In this regard, further research is required to validate the applicability of the trehalose regimen in the cryopreservation.
The present study demonstrated that sucrose and trehalose exhibited similar effects on ovarian histological structure, follicle ultrastructure, follicle number, and stromal cell density. However, trehalose presented additional advantages over sucrose in reducing ovarian cell apoptosis. Consequently, trehalose would be a more suitable cryoprotectant for intact ovaries in rats. These findings could facilitate further optimization of frozen transplantation technology for human intact ovaries, thereby potentially providing long-term benefits to female cancer patients and those with ovarian failure.
Data available on request from the authors.
LYX conducted the experiments, data analyzed, and wrote the main manuscript text; XYZ guided the study, data analyzed, prepared figures, and guided the manuscript text; KQH designed the study and guided the manuscript text; YD designed the study, conducted the experiments, wrote the main manuscript text and supplied funding. PX provided help and advice on material support and data analysis. All authors contributed to editorial changes in the manuscript. All authors read and approved the final manuscript.
The Ethics Review Committee of the Obstetrics and Gynecology Hospital of Fudan University approved the study (Grant NO.202209027Z). The use of animals in our experiments met ethical requirements. All activities related to this research project were performed in accordance with the relevant guidelines and regulations of the Obstetrics and Gynecology Hospital of Fudan University. The research protocol has been submitted to PreclinicalTrials.eu. Database (NO. PCTE0000461).
We appreciate the members in the Shanghai Key Laboratory of Female Reproductive Endocrine Related Diseases, and Obstetrics and Gynecology Hospital of Fudan University.
This study was supported by the National Natural Science Foundation of China (Grant NO.81901564).
The authors declare no conflict of interest.
Publisher’s Note: IMR Press stays neutral with regard to jurisdictional claims in published maps and institutional affiliations.