- Academic Editor
Background: By considering the importance and role of soil in the health of humanity, it is important to remove the presence of harmful compounds, such as phenol. Methods: In this study, four types of soil and leaf samples were collected from Kerman, Iran, and the amounts of heterotrophic and degradation bacteria were determined using the serial dilution and most probable number (MPN) methods. The amount of removed phenol was investigated using the Gibbs method with different concentrations of phenol. Then, an isolate with the highest percentage of phenol degradation was identified as the superior strain using 16 sRNA sequencing. The effects of the different factors, such as the carbon source (1% molasses and 1 g glucose), nitrogen source (0.1 g yeast extract), mixed culture, and time (14 and 28 days), on the biodegradation ability of the superior strain was investigated. Results: A total of 18 bacterial strains were isolated from the samples. Isolate B3 had the highest rate (75%) of phenol degradation, at a concentration of 1000 ppm, meaning it was identified as the superior strain. The molecular analysis results identified this isolate as the Comamonas testosteroni strain F4. This bacterium can degrade 89% of the phenol at 30 °C, 180 rpm, and 800 ppm over 28 days. C. testosteroni did not show a favorable phenol degradation ability in the presence of the investigated carbon sources, while this ability was also reduced in mixed cultures. Conclusions: C. testosteroni bacterial strain isolated from soil samples of pistachio orchards in Kerman, Iran, has a favorable ability to biodegrade phenol.
The global population is constantly increasing. Thus, as the population increases, the requirement to produce food also increases. In addition to the numerous roles soil plays in the ecosystem, it is also a valuable food source, providing 98% of human food [1]. In addition to changing the soil characteristics, which can make its conditions unfavorable, pollutants can enter living organisms from the water cycle or food and affect their survival and health [2]. For this reason, it is necessary to identify these pollutants in the water and soil sources and either remove or reduce them.
Previously, research has been conducted on various methods of removing or reducing environmental pollution, which also currently continues. In most cases, these methods are divided into two categories: physicochemical and biological. However, the disadvantages of physicochemical methods include high costs and the production of toxic compounds as byproducts [3]. Therefore, attention has shifted to the recognition and optimization of biological methods.
Biodegradation, biostimulation, and biological enhancement represent the current biological methods that use living organisms to remove or reduce pollution. During biodegradation, microorganisms use pollutant compounds as a source of energy, carbon, or nitrogen, and ultimately degrade them. If, due to some non-living environmental conditions, such as a reduction in some chemical factors occurs, biological decomposition by microorganisms becomes unachievable or slow, then, biostimulation is used instead. In this process, the addition of some nutrients and chemical compounds increases the ability of the microorganisms to decompose and function. Moreover, the biological enhancement of microorganisms with significant capabilities is used to eliminate or reduce contamination in an already contaminated environment. It seems that both methods of biostimulation and biological enhancement need more accuracy and investigation due to ecological changes, which lead to changes in the type and abundance of environmental microorganisms. Here, the use of native microorganisms is suggested in the contaminated areas in order to remove or reduce the contamination. However, some of the advantages of using biological methods over physicochemical methods are their greater environmental compatibility and cost-effectiveness [3, 4].
Phenol is an aromatic organic compound that is used in the production of phenolic resins, dyes, and synthetic textiles [5]. Phenol was one of the first compounds listed as a major pollutant by the US Environmental Protection Agency (EPA) [6]. Small amounts of it are dangerous and toxic for humans and other creatures, while chronic exposure to phenol by living organisms can be associated with problems in the digestive, nervous, skin, and reproductive systems. Degradation of phenol occurs both aerobically and anaerobically, however, it is not easy to decompose phenol [7, 8, 9]. For this reason, studies have been considered on the biodegradation of phenol by microorganisms. There are reports on the ability of bacteria and fungi to degrade phenol, of which the most researched bacterial strain is Pseudomonas [10].
The present research determines the abundance of phenol-degrading bacteria in soil and leaf samples and identifies the bacterial strain that possesses the optimal ability to remove phenol. In addition, some nutritional and environmental factors that potentially affect the bacterial strain’s ability to degrade phenol are tested.
Soil and leaf samples were collected from fields and gardens in Kerman province,
Iran, and investigated to isolate phenol-degrading bacteria. A total of 200 g was
collected from different places for each soil sample, from a depth of 0 to 10 cm,
and placed in sterile containers for transfer to the laboratory at a temperature
of 4
The total number of heterotrophic and degrading bacteria in the soil and leaf
samples was determined by the serial dilution agar plating method. Heterotrophs
were counted in each of the collected samples by preparing 10
Moreover, the most probable number (MPN) technique was used to count the total
number of heterotrophic and degrading bacteria in the leaf and soil samples.
Briefly, heterotrophs were counted by preparing 10
To isolate bacteria, 5 grams of each sample was added to 100 mL of BHB containing 0.02 g of phenol and incubated at 30 °C for 7 days with shaking at 160 rpm. The second passage was carried out using 5 mL of the suspension along with 100 mL of BHB medium containing 0.02 g of phenol as the carbon and energy source, implementing similar conditions as previously mentioned. Ultimately, 100 µL was cultured on NA medium and grown at 30 °C, after which, the different bacterial colonies were isolated [11].
The isolated bacteria were identified according to their known macroscopic and microscopic, biochemical, and carbon source characteristics depicted by Bergey’s Manual of Systematic Bacteriology [14].
For molecular identification, DNA was first extracted using the phenol–chloroform method before polymerase chain reaction was performed using a pair of 16 sRNA primers (Uni-1492R: TACGYTACCTTGTTACGACTT, Bac27-F: AGAGTTTGATCCTGGCTCAG) with time and temperature program of 95 °C for 5 min (1 cycle), 94 °C for 1 min, and 72 °C for 2 min (35 cycles), finishing at 72 °C for 10 min after the final cycle [15].
The amplification reaction was performed at a total volume of 25 µL, which
included 2 mM MgCl
PCR products were sequenced by Macrogen (Bio-Rad, Seoul, Korea) and a phylogenetic tree was constructed according to the neighbor-joining method by MEGA 4.1 software (Version 4.1.3, Zurich, Switzerland) [16].
The ability of the isolates to remove phenol was investigated using Gibbs reagent (2,6-dichloroquinone-4-chloroimide) [17]. A suspension of each bacterial isolate was prepared in a BHB medium with a phenol concentration of 0.02% or higher. For each concentration, a control sample was also considered without bacteria. The samples were examined after 7 days of incubation at 160 rpm and 30 °C by observing the blue color at 630 nm [18].
The growth rate and decomposition ability of the isolates were investigated at various concentrations of phenol, from 200 to 1000 ppm, while the isolate that indicated the highest growth and decomposition ability was again tested at higher phenol concentrations.
A bacterial pre-culture was grown in a BH medium with 200 ppm phenol, meaning bacteria could adapt to the phenol before testing at higher concentrations.
The effects of different factors of time, carbon source, nitrogen source, mixture of carbon, and nitrogen source on the isolate showing the highest ability to remove phenol were also investigated. The effect of time on the superior strain was investigated by inoculating bacteria in a BH medium with 800 ppm of phenol at 180 rpm and 30 °C on days 14 and 28. Two separate sources of carbon, molasses (1%) and glucose (1 g), were added to the BH with 800 ppm of phenol for 7 days, under the same conditions as mentioned earlier, to investigate the growth and decomposition of phenol by the determined superior strain. The effects of the nitrogen source and the effect of the carbon and nitrogen source mixture were investigated using 0.1 g of yeast extract, 1% molasses, and 0.1 g of yeast extract, respectively.
The results obtained from counting the bacteria in each sample are reported in Table 1. Based on the results shown here, using the serial dilution method, the highest number of heterotrophic bacteria was isolated from sample A and the lowest from sample C. Moreover, the highest number of decomposing bacteria was determined in sample B and the lowest was in sample D. According to the results of the MPN method, the highest number of heterotrophic bacteria was in samples C and D, whereas the lowest was in samples A and B. Moreover, the highest number of phenol-degrading bacteria was in samples A and B, whereas the lowest was in samples C and D.
Samples | Serial dilution | MPN | ||||
Heterotrophic bacteria | Degrading bacteria | Heterotrophic bacteria | Degrading bacteria | |||
In dilution 10 |
In dilution 10 |
In dilution 10 |
In dilution 10 |
|||
A | 7 × 10 |
8 × 10 |
9 × 10 |
7 × 10 |
2.015 × 10 |
4.2 × 10 |
B | 3 × 10 |
9 × 10 |
1.8 × 10 |
1.2 × 10 |
1.83 × 10 |
2.14 × 10 |
C | 4 × 10 |
6 × 10 |
6 × 10 |
8 × 10 |
4.5 × 10 |
1.1 × 10 |
D | 8 × 10 |
7 × 10 |
5 × 10 |
4 × 10 |
3 × 10 |
2.2 × 10 |
MPN, most probable number.
In this study, 18 bacterial isolates were isolated from the examined samples. All isolates were able to grow and degrade phenol at the initial concentration of 0.02 ppm, using it as the only source of carbon and energy. Most of the isolates used were coccobacilli and Gram-negative.
The phenol degradation and the growth rate of the isolates were investigated qualitatively and quantitatively. The highest OD values of 600 nm relate to four strains A2 (0.752), A3 (0.627), B3 (0.530), and B5 (0.501).
The results showed that three strains (A2, A3, and B3) could grow and degrade phenol at a concentration of 200 ppm (63%, 64%, and 54%, respectively). Therefore, these three strains were investigated at phenol concentrations of 400, 600, 800, 1000, and 1200 ppm (Fig. 1). The results showed that only the B3 strain could tolerate phenol at a concentration of 1200 ppm (47% degradation). Thus, the B3 strain was again tested using phenol concentrations of 1400, 1600, 1800, and 2000 ppm, while the degradation percentages at these concentrations were determined as 23, 12, 8, and 0, respectively. Subsequently, the B3 bacterial isolate was determined to be the superior strain, and a phenol concentration of 800 ppm was determined as the optimal concentration.
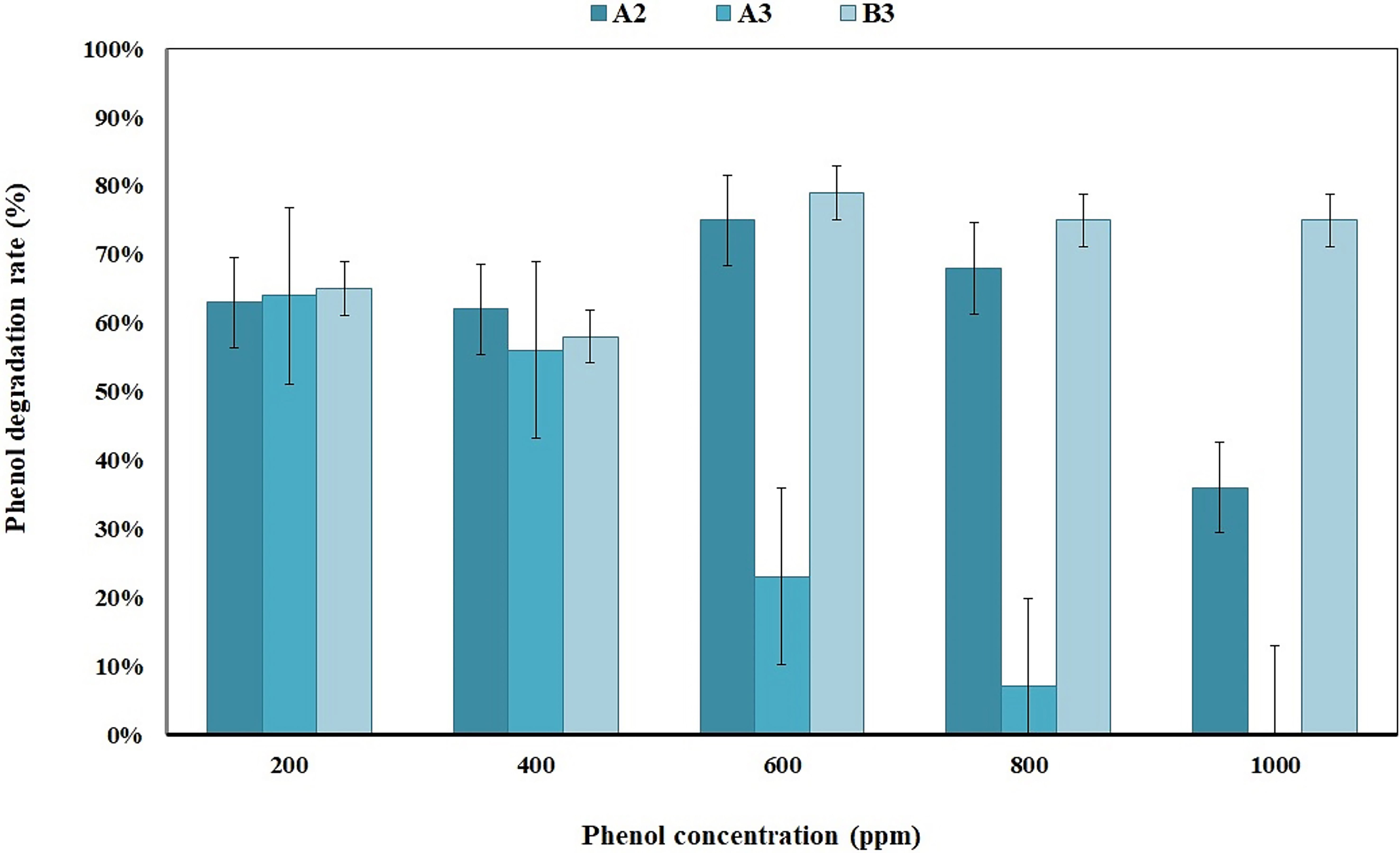
Phenol degradation of three bacterial isolates (A2, A3, and B3) in different phenol concentrations for an incubation period of 14 days.
The molecular analysis results for the B3 isolate indicate it as the Comamonas testosteroni strain F4 (Fig. 2).
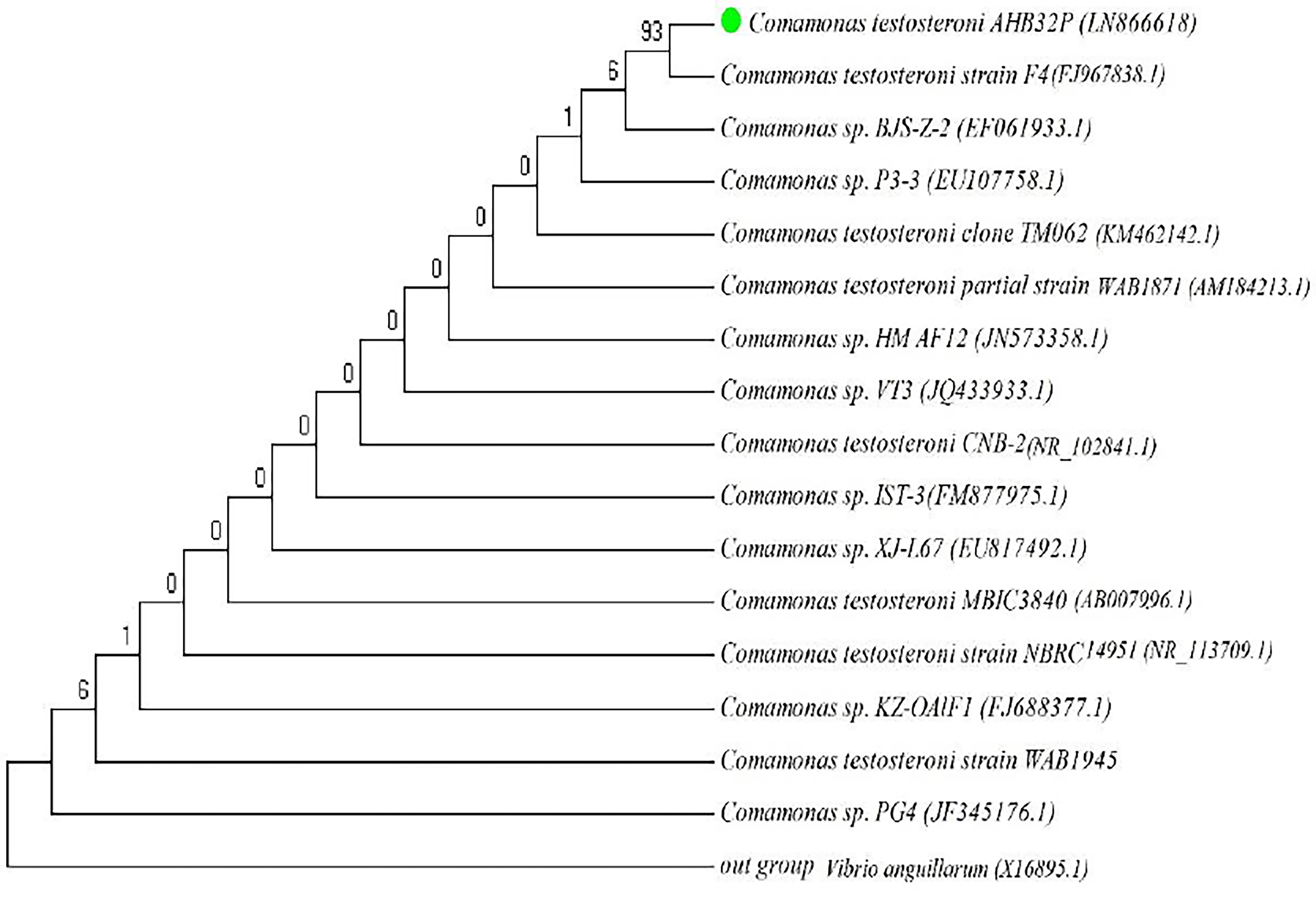
Phylogeny tree of isolate B3.
The effects of different factors on the degradation of phenol by B3 are shown in Table 2. The results show that B3 is unable to grow and degrade phenol (800 ppm) when using molasses and glucose as the carbon source. However, it can degrade phenol in the presence of yeast extract as the nitrogen source.
Investigated factors | Turbidity (OD 600 nm) | Phenol degradation (%) |
Carbon source (1% molasses) | 1.728 | 38 |
Carbon source (1 g glucose) | 1.159 | 33 |
Nitrogen source (0.1 g of yeast extract) | 0.830 | 53 |
Mixed culture (1% molasses + 0.1 g yeast extract) | 1.302 | 46 |
Time (14 days) | 0.860 | 54 |
Time (28 days) | 0.714 | 89 |
OD, Optical Density.
Currently, various types of research have been performed, which aimed to isolate and identify microorganisms with the ability to biodegrade phenol. Therefore, the methods and results obtained in this research can also be useful for a more comprehensive understanding of this research area.
The results of MPN and colony-forming units (CFUs) show that the type of soil
and sampling area are largely related to the microbial population of
heterotrophic bacteria by the amount of aromatic contamination, metal density,
and acidity. Many researchers have used CFUs to calculate bacterial densities.
Previous research conducted in a refinery by Esedafe et al. [19] showed
that the maximum amount of CFUs for heterotrophic bacteria in the soil sample was
2.7
In this research, the growth of the isolates and their ability to degrade phenol decreased as the phenol concentration increased. Thus, the B3 strain showed the highest phenol concentration tolerance among the 18 isolated strains. This result is probably due to the toxic effect of phenol in high concentrations of bacteria.
Putmai et al. [20] identified isolates of Proteobacteria and Actinobacteria that can decompose phenol and p-cresol in water, up to 500 ppm in 12 h. In another previous study, Panigrahy et al. [21] showed that the Pseudomonas citronellolis isolate could almost completely degrade 1500 mg/L of phenol in 90 h. The results of the current study showed that C. testosteroni decomposes 59% of the phenol at 30 °C, 180 rpm, and 800 ppm phenol over 28 days.
Tengku-Mazuki et al. [22] investigated salinity, temperature, and pH on the ability of Rhodococcus sp. (Gram-positive bacteria) to degrade phenol. The results indicated that the optimal conditions for phenol degradation of this bacterium are pH 7, 0.4 g/L NaCl, and 25 °C [22]. Moreover, some researchers investigated the ability of bacteria to degrade phenol in cold temperature conditions [23] or saline and hypersaline environments [24].
In the present study, the effects of different factors of carbon source, nitrogen source, and time were investigated on C. testosteroni bacterial isolates (Gram-negative bacteria). The results showed that time (28 days) produced a higher percentage of phenol degradation (59%) compared to the other investigated factors. Additionally, Ma et al. [25] performed a complete genome analysis on this bacterium and found that C. testosteroni bacteria have many genes for transportation. Moreover, many genes effective in the degradation of aromatic compounds were identified. However, due to the lack of protein-encoding genes involved in glycolysis and pentose phosphate pathways, this bacterium (C. testosteroni strain CNB-2) is unable to assimilate carbohydrates [25]. In the present study, C. testosteroni is not able to grow and degrade phenol in the presence of carbon sources: molasses and glucose, although it can degrade phenol in the presence of a nitrogen source (yeast extract).
Therefore, using a mixed culture of bacterial strains in the form of a consortium should also be considered. Strains that demonstrate a significant ability to degrade phenol in a single culture, if they are investigated as a mixed culture, affect the final efficiency of phenol degradation. In this study, the percentage of phenol removal by strains B3 and A2 at a concentration of 800 ppm was 75% and 68%, respectively. In the mixed culture of these two strains, the ability to remove phenol decreased by 49%. The study by Iheanacho et al. [26] showed that a consortium of Pseudomonas aeruginosa, Bacillus tequilensis, and Micrococcus sp. exhibited greater tolerance against the effects of phenol toxicity than individual isolates of each bacterium. The contradiction in these research results is probably due to the communication between these bacteria through different processes, including chemical and physiological, and therefore, requires more research and investigation.
Various types of soil, water, and sediment samples have been investigated to identify phenol-degrading microorganisms, which can be applied to reduce phenol pollution in the environment. In most cases, the results obtained in this research indicated that the C. testosteroni strain F4 bacteria isolated from the soil of pistachio orchards in Kerman, Iran, can be used to biodegrade phenol [27, 28, 29, 30, 31, 32, 33, 34, 35, 36, 37].
This study was performed to identify microorganisms that can degrade organophosphorus pesticides that are present in agricultural soils. These compounds have phenolic rings, which these bacteria can break to remediate the polluted soils.
The data will be available with request.
AM collected and analyzed the data; MH design the experiment; EA done statistically analysis; MNF, do experiment; AHA, and ALA, supervision the project and help for statistically analysis. All authors conceptualized and designed the research. AM collected and analyzed the data. All authors contributed to the interpretation of the data and writing of the manuscript. All authors have participated sufficiently in the work to take public responsibility for appropriate portions of the content and agreed to be accountable for all aspects of the work in ensuring that questions related to its accuracy or integrity. All authors read and approved the final manuscript. All authors contributed to editorial changes in the manuscript.
Not applicable.
The authors acknowledge the financial support from the Shahid Bahonar University of Kerman.
This work was supported through the Shahid Bahonar University of Kerman grants (Grant number 546987).
The authors declare no conflict of interest.
Publisher’s Note: IMR Press stays neutral with regard to jurisdictional claims in published maps and institutional affiliations.