- Academic Editor
Tempeh is an Indonesian traditional food made from fermented soybeans, which offers wide culinary use in East Asian countries. Similar to all fermented foods, its preparation offers the purpose of food preservation. However, preclinical studies have highlighted that microbial action leads to a modification in the nutritional composition of the food’s matrix. Although there is a wide availability of data on the beneficial effect of soy, tempeh remains relatively unexplored, perhaps due to its limited diffusion in the world, which limits its research availability. However, available data suggest that tempeh may confer beneficial health effects due to the high bioavailability of nutrients and phytochemicals, showing ameliorative action on oxidative stress, glycaemic control, and blood lipid levels. Furthermore, the high biological value of tempeh means it can be used to optimize protein and caloric intake in athletes, vegetarians, and children. Moreover, the microbial fermentation used in the production of tempeh, in addition to improving the bioavailability of minerals, proteins, fibre, vitamins, and isoflavones, produces biopeptides whose biological effect is currently of great interest. Tempeh can be employed in traditional preparations as well as second-generation foods, such as plant-based meat substitutes, to provide functional and nutritional properties and a higher eco-friendly option compared to animal foods. This review aims to provide an overview of tempeh’s properties, regarding human data and future research perspectives.
Ever since man has stabilized sedentary habits, the need for food conservation has become a fundamental role in daily food availability. Food fermentation consists of processing staples using microorganisms, with the main purpose of protecting against the growth of pathogenic microbes that cause food to spoil and pose health risks [1]. However, fermentation also allows the food matrix to be modified, providing different aromas from the starting food, by altering the bioavailability of nutrients and phytochemicals, improving nutritional quality, and conferring added functional properties [2, 3, 4]. Furthermore, the microorganisms being used for transformations can produce new molecules, such as vitamins, and by-products derived from microbial metabolism, including biopeptides with nutraceutical activities derived from matrix polypeptides, which have recently gained more interest for health purposes [5, 6].
There are different types of fermentation, which can be characterized by the presence of a selected starter, alongside the working conditions, and type of microorganisms being used. The most commonly employed microorganisms are yeasts, moulds, lactobacilli, and streptococci, which limit pathogen growth through acidification of the medium and microbial exclusion. Additionally, foods can be transformed by a heterogeneous pool of environmental microorganisms.
The use of fermenting plant foods is widespread throughout the world, with its roots in the culinary traditions of various countries, such as sourdough, sauerkraut, miso, tempeh, natto, kombucha, tamari, sufu, tofuyo, shoyu, kimchi, tape, doenjang, meju, douchi, cheonggukjang, kanjang, thua-nao, kinema, hawaijar, tungrymbai, oncom, etc. [1, 7, 8]. Many fermented foods from Southeast Asian countries extensively employ soy as a starting staple [9, 10, 11, 12].
Tempeh or tempe, as per its original name [13, 14], is a traditional Indonesian soybean food, which is obtained following various processes of dehulling, soaking, boiling, and fermentation [15, 16, 17]. During the last century, tempeh has spread to India, Japan, and the United States, more recently to Zambia in the early 1970s [15].
Multiple fermentation steps can be used during production, while treatments performed at different temperatures can follow one another along the production process. The use of various microorganisms may also vary based on the traditional use of environmental sources or through inoculations of specific isolated microorganisms. Even if there is a single decisive fermentation, other fermentation steps can occur to improve the product. Indeed, Fig. 1 displays the main steps in the soy-based tempeh production process.
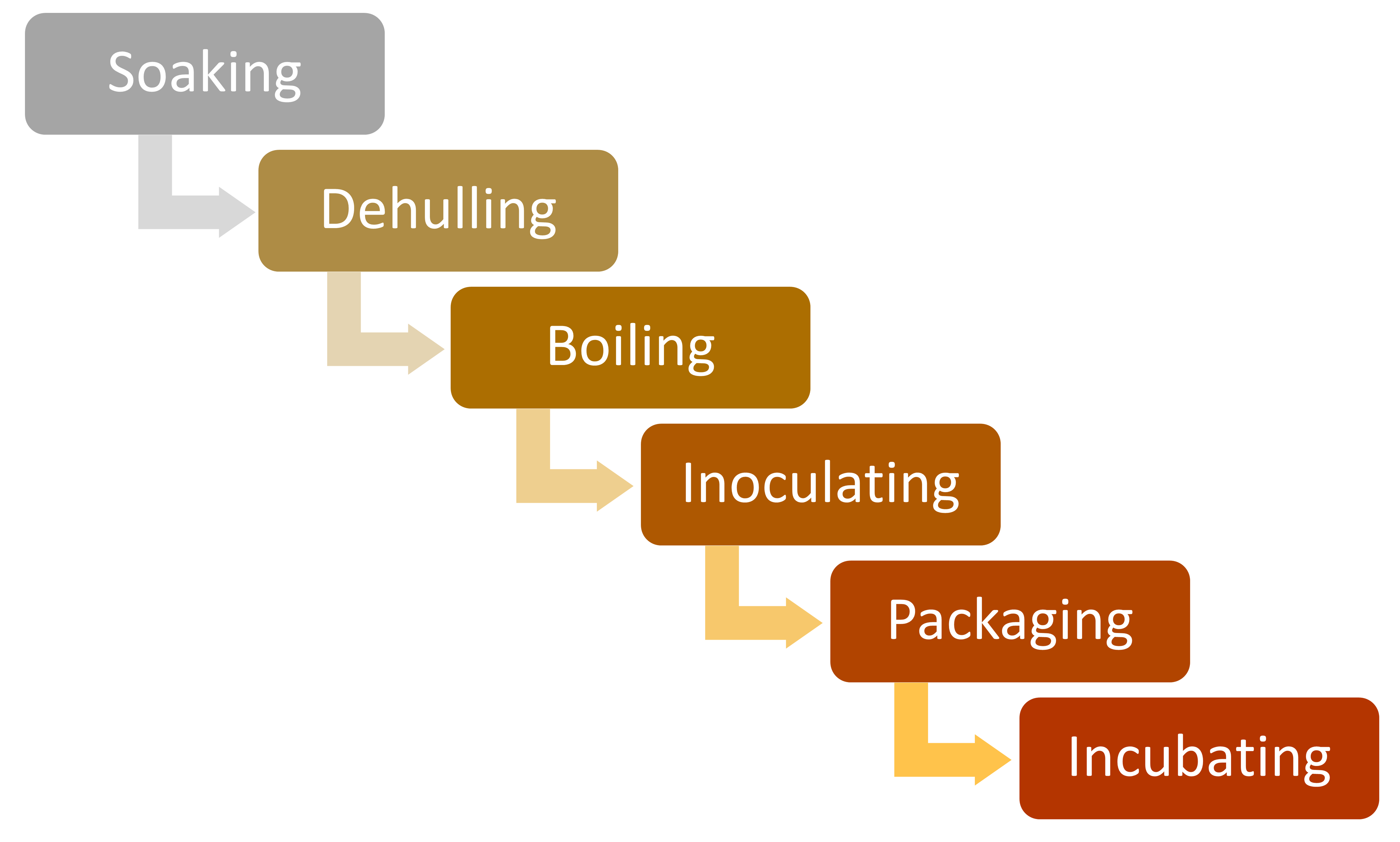
Production process of tempeh. The step order can change based on specific needs.
In Indonesia, tempeh is considered a cheap and affordable staple-based food, which is rich in proteins and can be consumed through a wide variety of cooking methods, including boiled, fried, grilled, or steamed [15]. Different types of rice, wheat, or brans are normally used as traditional inoculums. Heterogeneous pools of microorganisms can be found in traditional tempeh, with dominant strains such as S. dysgalactiae, S. faecium, S. epidermidis, and L. casei identified in the soaking step. Likewise, B. brevis, C. diversus, E. agglomerans, E. cloacae, K. pneumoniae, K. ozaenae, P. burtonii, R. rubra, and C. diddensiae have also been detected [18]. The use of Lactiplantibacillus plantarium (formerly known as Lactobacillus plantarium and L. arabinosus) during the soaking step seems to favour the acidification medium, which inhibits the growth of various pathogenic microbes [19, 20, 21, 22]. In the industrial production of tempeh, the fermentation step is conducted by packing soaked and inoculated soybeans into perforated plastic bags. However, naturally available materials are used during the traditional fermentation process. Fig. 2 shows a finished traditional tempeh product, whereby Hibiscus tiliaceus leaves are usually used for packing inoculated soybeans before the fermentation step.
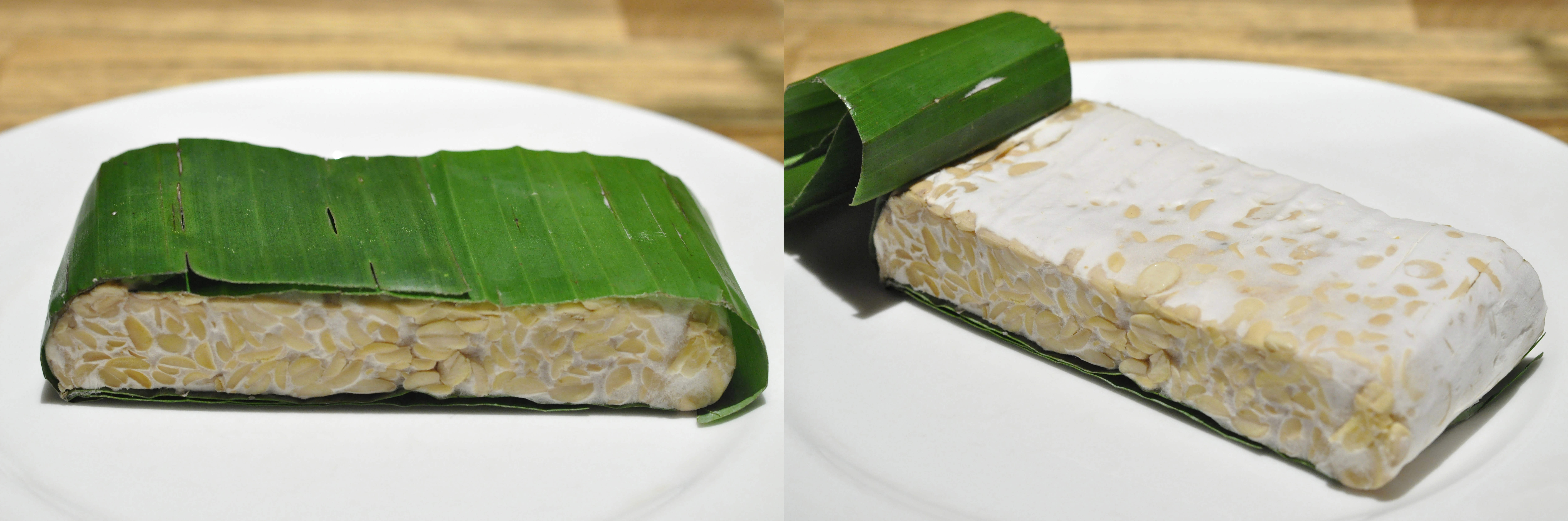
Traditional tempeh. Hibiscus leaves are used to package inoculated soybeans.
A single species of filamentous fungus is used in the fermentation step, with the common employment of Rhizopus species, such as R. oligosporus, R. oryzae, R. stolonifer, R. arrhizus, and R. formosaensis, alongside other microorganisms belonging to Fusarium spp [23, 24]. Among these moulds, Rhizopus microsporus var. oligosporus is the most used globally for producing tempeh [25]. However, other microorganisms such as bacteria, moulds, and yeasts may be present in traditional co-inoculums, such as Absidia spp., Mucor spp., Rhizomucor spp., etc. [26]. In non-traditional co-inoculations, probiotics such as L. plantarium have been added to the main fermentation step, which can greatly inhibit the growth of pathogens during this phase [22]. The co-inoculation of B. subtilis, a bacterium used in the production of Natto, can also confer additional functional properties to tempeh [27], while the use of A. elegans can reduce the presence of non-digestible oligosaccharides that produce flatulence [28].
Solid-state fermentation changes the consistency of the starting matrix, producing a cake-formed product with a white covering texture by the mycelium, which compacts the tempeh structure and causes a mushroom-like flavour [29, 30]. Although soy-based tempeh is the most widespread, the same transformation process can be applied to various legumes and grains [31].
As with many other fermented foods, the production process reduces the concentration of antinutrients in the food matrix (such as protease inhibitors, tannins, and phytic acid), thereby causing an increase in the nutritional value of the product compared to the starting food [3].
Although the properties of tempeh have been described in preclinical studies, human studies are still very fragmentary if we consider the large availability of studies on soy [32, 33, 34, 35, 36, 37, 38, 39].
This review aims to describe the possible benefits of soy-based tempeh for humans, collecting available data from previously published literature. This document provided a comprehensive update of the current knowledge on biotechnology and biochemical characteristics of soy-based tempeh, with particular attention on human outcomes and benefits related to its use. Although previous reviews on the topic are available, the information proposed is frequently based on animal and in vitro studies, with marginal parts regarding the benefits on humans. A relevant part of this document was dedicated to nutritional values, nutraceutical properties, and current health concepts, such as postbiotic and paraprobiotic actions of secondary metabolites from fermented food. Moreover, well-known bioactive molecules with health benefits for metabolic diseases are also discussed.
Tempeh is a food low in carbohydrates and rich in proteins, vitamins, and bioactive compounds [30]. Fermentation allows the release of free fatty acids from lipid fractions [40] and transforms soy glycosidic isoflavones into unconjugated aglycone [41, 42, 43]. According to the Codex Alimentarius Commission, the protein component must represent at least 15% w/w with a lipid content of 7% and dietary fibres representing at least 2.5% [29]. According to the United States Department of Agriculture Standard Reference (USDA SR) Legacy Foods database, tempeh provides 192 kcal per 100 g, 20.3% protein, 10.8% fat, and 7.64% carbohydrates [44]. Furthermore, 100 g of tempeh consists of 111 mg of calcium, 2.7 mg of iron, and 24 mcg of folate. Fermentation leads to an increase in iron bioavailability owing to the conversion of iron (III) into iron (II) [45, 46]. Furthermore, there are 4.3 g of polyunsaturated fatty acids per 100 g, the majority of which is represented by linoleic acid. This polyunsaturated fatty acid is an essential molecule in the omega-6 series that is particularly represented in soy and its derivatives [47]. In the USDA-branded foods database, a very similar composition can be found, with frequent use of white, brown, or wild rice among ingredients. Nutritional composition among different batches and preparations of tempeh can vary according to the microbial species being used and the fermentation process conditions, such as time and temperature. Table 1 shows the nutritional composition of tempeh according to the USDA SR Legacy Foods and Branded Foods database.
Product | Energy (kcal) | Protein (g) | Fat (g) | Carbohydrate (g) | Fibre (g) | Calcium (mg) | Iron (mg) | Ingredients |
USDA SR Legacy Database | 192 | 20.3 | 10.8 | 7.64 | NA | 111 | 2.7 | |
Lightlife (a) | 190 | 21.4 | 5.36 | 14.3 | 7.1 | 104 | 2.38 | Cultured organic soybeans (soybeans, lactic acid from plant source), water, and organic brown rice |
Nature’s Promise | 224 | 23.5 | 9.41 | 12.9 | 4.7 | 94 | 1.65 | Cultured organic soybean and water |
Lightlife (b) | 202 | 16.7 | 5.95 | 21.4 | 8.3 | 71 | 2.14 | Cultured organic soybeans (soybeans, lactic acid from plant sources), water, organic wild rice, and organic brown rice |
Franklin Farms | 227 | 22.7 | 9.33 | 13.3 | 5.3 | 93 | 1.73 | Cultured organic soybean and water |
Westsoy | 211 | 21 | 9.21 | 11.8 | 3.9 | 105 | 2.37 | Cultured organic soybean, water, and organic white rice |
A protein-energy deficiency persists in low-income countries, caused by low accessibility to food in many parts of the world. Furthermore, plant foods may have limited protein value due to their low amino acid score and digestibility. Soy is a well-known food source that is rich in essential amino acids. However, the digestibility of soybeans is limited by the presence of antinutrient substances commonly found in legumes, such as phytic acid, tannins, and protease inhibitors. There is a lowering of phytic acid concentrations during microbial fermentation and soaking, [46], which improves the bioavailability of proteins owing to an increase in digestibility caused by a reduction of antinutrients. Although the overall amount of protein is very similar to unfermented soybeans, tempeh offers better protein quality. Fermentation increases the protein digestibility corrected amino acid score (PDCAAS) compared to the starting pulse [48]. The PDCAAS is a parameter that is widely used in the evaluation of protein quality since it considers both the chemical composition of amino acids and the physiological absorption of proteins. Furthermore, microbial degradation of proteins by microorganisms releases free amino acids into the matrix, which has possible functional implications [49].
Tempeh is considered a rich source of branched-chain amino acids (leucine, isoleucine, and valine) due to the increase in their bioavailability after fermentation [50]. Moreover, the fermentation process can slightly increase essential amino acid bioavailability compared with the starting staple [51]. The high protein quality of tempeh can be useful for ensuring affordable vegetable protein sources with high nutritional values. Furthermore, these characteristics are often attractive to those who follow either a vegetarian or vegan diet and are looking for alternative nutritional sources. Considering that a plant-based diet can be adopted for ethical, health, and environmental reasons [52, 53], tempeh can be highly accepted among vegetarians and vegans.
Vitamin B12 is an essential molecule for humans. It takes part in mammalian metabolic pathways as an enzymatic co-factor in metabolising odd-chain fatty acids and for homocysteine detoxification [54, 55]. It is considered a vitamin of animal origin since the concentrations in plant foods are negligible. However, its biosynthesis is carried out by microorganisms, meaning fermented foods can contain vitamin B12 if competent microbes are present [56, 57]. Based on the microbial consortium composition of tempeh fermentation, significant quantities of vitamin B12 derived from symbionts can be found [58, 59]. The production of vitamin B12 in tempeh usually depends on the presence of K. pneumoniae and C. freundii in the microbial consortium [58, 60, 61]. It seems that the main mould used for fermentation does not contribute to vitamin B12 production. Generally, the presence of this vitamin is dependent on contaminating and causal events and it can cause up to 12.4 mcg of vitamin B12 per 100 g of traditional tempeh [58]. In countries of traditional production, where natural water sources are used, environmental bacteria can synthetize vitamin B12 [58]. However, a cross-feeding network appears to exist between vitamin B12-producing species and Rhizopus [61]. Moreover, Propionibacterium species can be useful in increasing vitamin B12 concentrations in tempeh batches and they could be industrially adopted for this purpose [62, 63].
Since the availability of vitamin B12 in plant-based foods is negligible among vegetarians, the possibility of frequently using tempeh in steady amounts could help to guarantee dietary vitamin B12 requirements [57]. However, considering that concentrations are still not standardized by the various producers, and tempeh is a food option not often consumed daily in countries different from the places of traditional production, it remains premature to confer this nutritional role to tempeh.
Although vitamin B12 is of great interest due to its limited availability in nature, the fermentative action of microorganisms can promote the bioavailability of other vitamins. Co-inoculation of tempeh with Aspergillus oryzae compared to R. oligosporus alone shows a considerable increase in vitamin B1 and vitamin B2 levels, although it has been detected in grass pea and not soybean [64]. The increase in vitamin B3 both in the form of nicotinamide and nicotinic acid has been observed in batches of tempeh fermented with C. freundii and Lactobacillus spp., while vitamin B1 can be produced by C. freudnii [65]. The fermenting microbes increased vitamin B8 content by more than 2 times compared to the basic matrix [66]. Folates also seem to increase from fermentation, while the content in tempeh can increase five-fold compared to the starting food [66, 67, 68]. Tempeh has also been identified as a source of ergosterol, a provitamin isoform of calciferol called vitamin D2, which is biosynthesized by fungi, including microbial [69, 70].
As already mentioned, the reduction of antinutrients through the fermentative transformation process is a crucial step in increasing the soy nutrients bioavailability found in tempeh, in particular, the polyphenols, minerals, and proteins [3]. Although the cooking and soaking steps contribute toward the reduction in phytic acid, fermentation appears to be the most effective step for this activity [3, 30]. This is possible thanks to the action of thermostable phytases of R.oligosporus, which work at an optimum temperature of 44 °C and pH of 3–5 [71, 72]. R. oligosporus phytases reduce the phytic acid content by approximately 65% [73]. Some lactic acid bacteria can also show phytase activity [72, 74]. Similarly, oxalate levels in tempeh appear to be lower than in other plant foods, including textured soy protein, which shows levels that are almost 28 times higher [75]. The levels of trypsin inhibitors are also reduced in tempeh following fermentation, which also leads to improved protein digestibility [45].
Stachyose, raffinose, and alpha-galactooligosaccharides are all reduced by the fermentative action of the microbes found in tempeh, thereby reducing flatulence resulting from indigestible oligosaccharides [73, 76, 77, 78]. Moreover, regarding allergenicity, the effect of fermentation allows allergens hydrolysis to occur, which also happens in other fermented foods [79, 80]. This aspect can be further enhanced by selected co-inoculations that favour the protein hydrolysis from the matrix [28]. A further improvement in protein quality occurs through the reduction in phytates and indigestible oligosaccharides as well as an increase in antioxidant power, which was obtained by germinating the soybeans used for tempeh production [81, 82, 83].
Tempeh, unlike soybeans and other legumes, is among the foods listed by Monash University resources as low in fermentable oligosaccharides, disaccharides, monosaccharides, and polyols (FODMAPs) [84, 85].
Fermentation increases soluble iron from 24% to 67% owing to the release from the vegetal ferritin soy deposits, probably due to the phytase activity [13]. The increase in iron bioavailability also depends on the conversion of iron (III) into iron (II) [46, 86].
It has been suggested that soy products may provide a potentially beneficial effect on bone health due to their isoflavone content [87, 88, 89]. Furthermore, microbial fermentation in tempeh also improves calcium bioavailability, which may contribute to improved bone density [13]. Haron and colleagues [90] evaluated calcium absorption from tempeh in 20 post-menopausal Malaysian women and compared it to cow milk in a cross-over clinical trial. Following the intake of approximately 200 g of tempeh, compared to 114 grams of milk (containing the same amount of calcium), no significant differences were noted in food-dependent absorption, thereby indicating that tempeh may represent a bioavailable plant-based source of calcium, with fractional absorption of calcium similar to milk. Furthermore, urinary calcium excretion was not significantly different, although calcium balance was higher for tempeh compared to milk.
The antioxidant effect of tempeh has been verified in vitro and is mostly associated with the presence of isoflavones, such as genistein and in particular aglyconic forms, in addition to the potential effect of trans-cinnamic acid [91, 92]. However, soy contains non-isoflavone polyphenols, including lignans—also classified as phytoestrogens—which can contribute to the antioxidant effect of tempeh [93].
The use of L. plantarium as a co-inoculum in tempeh production favours the reduction of biogenic amines, such as putrescine and tyramine, which can be formed due to the growth of unwanted microorganisms [94]. The strains used in this production can be decisive in obtaining various functional properties related to tempeh since the microorganisms specific proteolytic activity can release the biopeptides responsible for some antioxidant, anticancer, antidiabetic, and antihypertensive properties by tempeh [95]. The action of isoflavones could also influence cholesterol levels [96, 97]. It also appears that free fatty acids from tempeh exert a cholesterol-lowering effect by inhibiting hepatic 3-hydroxy-3-methyl-glutaryl-coenzyme A (HMG-CoA) reductase [98]. Furthermore, the hypocholesterolemic effect could depend on the cholesterol intestinal sequestration effect of enterohepatic circulation by dietary fibre, while reducing the glycaemic response by inhibiting the absorption of macromolecules [99, 100, 101]. Tempeh has shown anti-aging effects in pre- and post-menopausal women, as assessed by skin quality, bone density, and uterine tissue health [102, 103]. These mechanisms appear to be related to the interaction between isoflavones and the female hormone network [104]. Furthermore, the oestrogen-like activity of isoflavones has been proposed to provide anticancer effects [105, 106].
Soy isoflavones are widely studied for their potential beneficial effects. They are polyphenolic phytochemicals that provide strong antioxidant effects [32]. However, they are much better known for their interaction with oestrogen receptors that produce differential activity between the ER-alpha and ER-beta; therefore, promoting their tissue-specific and oestrogen receptor-modulating effects [107, 108, 109]. Isoflavones can interact with oestrogen receptors thanks to their molecular structure, which resembles endogenous oestradiol and which, together with lignans and coumestans, is classified as a phytoestrogen due to these characteristics [33, 110]. Even if some previous preclinical studies have presented a possible negative endocrine effect of phytoestrogens, presently, we know that isoflavones can exert a beneficial effect on the human hormonal network, stimulating oestrogen receptors when circulating endogenous oestrogens are insufficient, and concurrently, competing with circulating oestrogens when their concentrations are high, thereby mitigating their overall effects [34]. For this reason, isoflavones, including soy-derived phytoestrogens, are used to mitigate menopause nuisances.
Although phytoestrogens are present in many foods, such as seeds, legumes, and other vegetables, the main nutritional sources of isoflavones are soy and soy-based foods. Isoflavones such as genistein, daidzein, and glycitein are found in soybeans in glycosylated forms as malonyl and acetyl compounds conjugated to sugars. However, these isoforms appear to be poorly bioavailable due to limited diffusion through the intestinal mucosa [111]. Many soy foods contain mostly isoflavones in a glycoside form. Some soy processing methods allow isoflavones to be metabolised, transforming them into aglycone, which diffuses more easily through lipid membranes thanks to their lower hydrophilicity and molecular weight, compared to their respective glycosides [112, 113, 114, 115]. Fermentation during tempeh production is widely documented as being able to perform this transformation and, therefore, confer greater benefits from isoflavones resulting from the increase in their bioavailability after deconjugation [41]. The percentage of aglycone in fermented foods varies from 40% to 100% as a result of the microbial beta-glucosidase action. Furthermore, the isoflavone content in traditional foods, such as tofu and soy milk is on average higher than in second-generation soy products [112]. However, tempeh appears to be the soy-derived food with the highest levels of isoflavones [116]. Various production steps can favour the deconjugation of isoflavone, while the fermentation time can also affect the final isoflavone levels [117, 118, 119, 120]. Even frying the tempeh (one of the most traditional cooking methods) appears to further improve the concentration of aglycone isoflavones compared to raw tempeh, although it simultaneously reduces the total isoflavones content [121, 122]. Pre-germination of soybeans appears to further increase the isoflavones bioavailability by releasing the germinated isoflavone content [123].
Equol represents the most active form of daidzein because its structure is very similar to endogenous 17-beta-estradiol and its presence in circulating fluids is closely linked to the individual ability of metabolising isoflavones [106]. In addition to the greater estrogenic effect, it also shows a greater antioxidant effect compared to daidzein [124, 125, 126, 127]. Although clinical trial data are conflicting as to which soy foods (tempeh, soymilk, or texturized soy protein) are more effective in raising genistein or daidzein levels in the urine following intake [128, 129], major equol concentrations have been found following the consumption of tempeh [128]. However, data are still insufficient to definitively clarify these aspects. Equol and O-desmethylangolensin (O-DMA) represent the products of daidzein intestinal metabolism after its transformation into the aglyconic form [130]. Nevertheless, since this step is performed by the intestinal microflora, there may be individual variability in the ability to produce equol [131, 132, 133]. Some individuals are defined as equol-competent, equol-producer, or equol-excreter if they excrete higher concentrations of equol following daidzein intake [130, 134, 135, 136, 137]. It is estimated that in the general population, only 30% of individuals are equol-producers [138, 139, 140, 141]. This also implies that individuals who are unable to metabolize isoflavones due to a lack of adequate intestinal microbial populations may not fully benefit from the activities of isoflavone [134, 142]. In mammals, isoflavones follow the enterohepatic circulation, meaning they are excreted in a conjugated form to glucuronic acids by bile [143]. Subsequently, the isoflavones can be deconjugated again by bacterial beta-glucuronidases and sulfatases. Therefore, the gut metabolism of isoflavones is a complex biochemical process, whereby both the host and microbial enzymes participate [144, 145, 146].
In 20 post-menopausal Malayan women, tempeh consumption resulted in greater urinary excretion of equol, daidzein, and genistein compared to milk consumption [147]. In one of the first works on the bioavailability of phytoestrogens from fermented soy foods, Hutchins and colleagues highlighted how fermentation during tempeh production reduces the content of total isoflavones, especially those in the glycosidic form (daidzin and genistin), yet it increases the recovery of isoflavones in urine following ingestion due to its increased bioavailability [148]. However, in a study by Xu and colleagues [149], on 10 healthy fertile women, the consumption of various types of soy foods (tempeh, tofu, textured soy, or soybeans) caused no significant differences in short-term urinary isoflavone excretion. However, the limited statistical power of these clinical trials makes it difficult to conclude an overall outcome, also considering the influence of interindividual variability on isoflavone metabolism. Subsequently, a clinical trial was performed with 27 men and women (both pre and post-menopausal), which tested the urinary kinetics of acute isoflavone intake using soy milk, tempeh, and textured soy proteins [128]. Recovery of urinary genistein was greater in tempeh for premenopausal women than by soy milk, although this was not observed in men and postmenopausal women. While there were no appreciable differences in daidzein excretion related to the food matrix, consumption of tempeh showed greater excretion of equol compared to other soy foods. Similarly, Cassidy and colleagues [129] enrolled 59 individuals to investigate the pharmacokinetics of isoflavones from various soy foods: tempeh, soy milk, and texturized soy. Following the ingestion of tempeh, there was a greater increase in serum daidzein and genistein compared to the other soy foods. However, the blood concentration of isoflavones peaked faster following the consumption of soy milk, thereby highlighting the favourable influence of a liquid matrix compared to the solid state. Although the highest concentration of total isoflavones was found in texturized soy proteins, tempeh produced the highest concentrations of genistein and daidzein in aglyconic form.
With the increase in the average lifespan of the population, the occurrence of cognitive disorders has also increased, which results in greater healthcare costs. Currently, the clinical approach to cognitive decline is palliative, with the aim of slowing down the disorder. Lifestyle approaches such as physical activity and nutrition remain the most promising preventive interventions. However, meta-analyses have highlighted a positive effect of oestrogen administration on cognitive test outcomes for up to 2–3 months [150, 151]. Furthermore, the effects on cognitive functions from modifying the microbiota appear to involve various mechanisms [152, 153, 154].
In an observational study on 142 elderly individuals from rural Indonesia (56–97 years old), it emerged that tempeh consumption was correlated with better immediate recall scores [155]. More recently, Handajani and colleagues [156] conducted an intervention study on 84 participants aged over 60 years with mild cognitive impairment (MCI), who had been randomized to consume two different tempeh preparations compared to a control group. After 6 months of consuming 100 g of tempeh per day, there was an increase in global cognitive scores in participants who had assumed tempeh, although an improvement in language domain scores was only associated with one of the two preparations. Interestingly, the effective preparation for the language domain was the preparation with the lowest concentration of microorganisms, indicating that the qualitative aspect rather than the quantitative one may be more important. A probiotic strain of L. reuteri fermentum isolated from tempeh was used for a 12-week clinical trial on 93 Indonesian individuals over 60 years old with memory impairment [157]. From the 16S rRNA analysis, the selected strain contained the gene encoding for gamma-aminobutyric acid (GABA). The intervention improved the values in the cognitive domains of memory, learning processes, and verbal and visuospatial fluency.
This suggests that tempeh consumption may be useful for a safe intervention in cognitive preservation, although only during a still receptive age window.
A drink with added tempeh extract was used in a double-blind controlled clinical trial on 18 semi-trained men aged 18–24 years to evaluate parameters associated with muscle tissue damage and recovery [158]. The tempeh drink significantly reduced creatine kinase levels and increased maximal muscle strength at 24 hours compared to the placebo. These effects showed no significant differences compared to the whey protein control group. Creatine kinase levels were linked to muscle damage and indirectly to muscle fatigue, while also reducing muscle strength and recovery [159]. Therefore, tempeh with its antioxidant properties could mitigate the effects of reactive oxygen species (ROS) produced by excessive training, which can damage muscle cell membranes [160, 161].
In addition to these sport-related benefits, the nutritional characteristics of tempeh could be applied to improve calorie-energy adequacy, especially in children. In a recent study, tempeh was provided as nuggets to 46 Indonesian children aged 24–59 months [162]. After 30 days, tempeh consumption had improved energy intake compared to the control. Similarly, 30 g/day of tempeh nuggets were offered to 30 children aged 36–60 months in a quasi-experimental study and showed a significant weight increase after 4 weeks [163]. In contrast, the control showed a non-significant weight gain compared to baseline.
Tempeh, given the effect of fermentation, can be a highly digestible food for children [1, 164]. Moreover, it does not result in an excessive intake of fibre, which in the case of a vegetarian or vegan diet could represent a limit in the absorption of nutrients by children [165]. The use of tempeh as an adequate nutritional source in children is a promising frontier, which would allow suitable economic solutions for low-income countries to address calorie-energy insufficiency. At the same time, as a minimally processed source of legumes, it would help the objective of the FAO to increase the consumption of legumes, as an eco-friendly food security strategy, while also limiting food excesses in high-income countries [166, 167]. Given the high environmental impact of the animal food production chain (water use, carbon footprint, and greenhouse gas emission), the use of plant-based foods with high nutritional value can encourage a transition toward a more plant-based diet, guaranteeing greater access to food and reducing the ecological cost of production.
In a prospective, open-label intervention study on 35 Indonesian patients with type 2 diabetes, daily consumption of 2 grams of tempeh for 3 months improved HbA1C and triglyceride levels [168]. However, since this trial used a freeze-dried sample of tempeh grounded into a powder, it is not possible to transfer the results to cases where fresh tempeh was consumed, which is normally cooked prior to consumption. In a randomized, double-blind controlled, cross-over trial, 13 Indonesian women aged 18–20 with a BMI of 25–30 used tempeh or unfermented soy for an acute test [169]. A 30% reduction was observed in serum acyl-ghrelin 30 minutes after ingesting tempeh, yet not following unfermented soy consumption. Furthermore, the fermented food showed a greater insulin response and an increase in serum arginine at 120 min compared to the control. Ghrelin is considered the hunger hormone, and it is produced by gastric cells during fasting, while it is reduced following the ingestion of a meal [170, 171]. Similarly, insulin is released after a meal by pancreatic cells to stimulate satiety [172]. Furthermore, arginine is an amino acid that is released during protein digestion, which could act as a signal of meal protein sufficiency [173]. This suggests that tempeh could improve the ability to regulate appetite, which may be beneficial in individuals with metabolic syndrome.
Tempeh may have antimicrobial properties that can positively influence the balance of intestinal microbiota following ingestion. A liquid suspension of tempeh was able to inhibit the growth of B. subtilis and B. stearothermophilus in vitro [174, 175]. R. oligosporus can inhibit the growth of Bacillus spp, Staphylococcus spp and Streptococcus spp. through heat-resistant proteins with antimicrobial activity being released into the growth medium [176].
This aspect is particularly relevant since tempeh is consumed after cooking, meaning a probiotic effect seems unlikely. However, the substances released following fermentation, if resistant to cooking, can exert a paraprobiotic effect, which modulates the intestinal microbiota ecology [35].
An in vitro study that simulated human gastrointestinal digestion highlighted how tempeh can stimulate the levels of Bifidobacterium spp. and Lactobacillus spp. [175]. Akkermasia muciniphila together with Bifidobacterium spp. is more represented in the gut of lean individuals rather than in subjects with metabolic syndrome [177, 178, 179]. A. muciniphila colonizes the cecum and degrades the glycoproteins that make up the mucin, thereby stimulating the immune system and cell proliferation in the host mucosa [180]. In an open-label study on 16 participants, consuming 100 g of steamed tempeh for 16 days increased A. muciniphila numbers and immunoglobulin A (IgA) levels in the stool compared to the baseline [181]. IgA acts as a first defence against pathogens and toxins in the intestinal tract and promotes anti-inflammatory mechanisms that shape the intestinal barrier through the microbial balance of the microbiota [182].
Subsequently, Stephanie and colleagues confirmed an increase in A. muciniphila in the faeces after 28 days, also showing an increase in Bifidobacterium spp. compared to the baseline [183].
The effectiveness of tempeh in this clinical trial confirms the paraprobiotic effect since cooking before consumption inevitably destroyed the present microorganisms, which cannot act through a probiotic mechanism. However, the molecular motifs remaining from the microorganisms present in fermented food can be recognized by the host’s immune system and they activate a response following the antigenic interaction [184]. Furthermore, the presence of fermentable fibres in tempeh could favour the microbial production of short-chain fatty acids (SCFAs: acetate, butyrate, propionate), which show a beneficial effect in the gut by exerting a prebiotic effect [185]. Moreover, SCFAs can exert anti-inflammatory and hypocholesterolemic actions on the host by inhibiting the hepatic synthesis of HMG-CoA reductase [99, 101, 186]. The production of SCFAs has also been linked to gut–brain axis (GBA) functions [187, 188].
Depending on the fermentation conditions, GABA was found in tempeh [189]. GABA is a neurotransmitter implicated in cognitive functions, of particular interest in GBA communication mechanisms [190, 191, 192].
The antioxidant effect of tempeh components seems to be related to fermentation times with greater ROS scavenging activity following prolonged fermentation [193, 194, 195]. In vitro studies have shown that tempeh has a greater antioxidant effect than unfermented soy, showing greater scavenging abilities on free radicals and superoxide anions [194]. In a recent in vitro study, microglial cells showed reduced ROS levels following tempeh administration after lipopolysaccharide (LPS)-induced oxidative stress [196]. This may depend on the content of polyphenols and their bioavailability [41, 193, 197]. The presence of greater isoflavone concentrations in the aglycone form seems to explain the properties of tempeh compared to other non-fermented soy foods and it identifies genistein and daidzein in the aglycone isoforms as having greater antioxidant effects compared to their glycosides, as well as a greater bioavailability [198, 199, 200]. Furthermore, microbial proteolytic activity allows proteins to be hydrolysed and release amino acids and biopeptides, which are more bioavailable and exert antioxidant activities [201, 202].
The increase in antioxidant activity by tempeh may also depend on the increase in phytochemicals as is the case for beta-tocopherol, which shows a 200% increase in activity compared to unfermented soybean [203]. While beta-, gamma-, and delta-tocopherol concentrations seem to increase during tempeh production, the concentration of alpha-tocopherol is not affected by the fermentation process.
Another powerful antioxidant identified in tempeh is 3-hydroxyanthanilic acid [204]. This showed an improvement in stabilizing soy products through the scavenging effect of NO [204, 205]. The antioxidant power can be further increased by including an anaerobic fermentation step in tempeh production [206]. The action of oxidative stress appears to be related to numerous chronic pathologies, such as diabetes, inflammatory diseases, tumours, and cardiovascular [16, 207, 208, 209].
The bioactive molecules contained in tempeh, in particular biopeptides, can exert a beneficial effect on cancer prevention through a protective antioxidant effect on nucleic acids. The same beneficial effect can be advantageous in cancer therapy. However, to obtain the maximum benefit from consuming tempeh it may be useful to preserve the microbial component by limiting transformations such as deep cooking and its transformation into flour [210].
The consistency of tempeh and its nutritional properties make it ideal for use in second-generation foods, such as meat substitutes, which are highly requested in Western countries [162, 211, 212]. Furthermore, tempeh-based preparations can retain functional properties compared to meat-based foods [213]. Tempeh can also be used as a meat extender to improve the nutritional properties of meat-based preparations and at the same time limit production costs [214, 215]. Similarly, the use of tempeh in mixtures for pasta production allows for an increase in the content of folates and proteins, while also improving the taste and texture [216, 217].
Acceptability and increase in polyunsaturated fatty acid content were found when 5% dehydrated tempeh was used in bread doughs and cereal-based bars [218]. The antioxidant capacity is preserved even when employed for the production of biscuits as a replacement for soy flour components [219]. This use does not alter the product acceptability. Tempeh has also been used as a fortifier to obtain an emergency food formula [220].
The use of tempeh in doughs has been successfully used for the production of vegan biscuits to increase acceptability [221]. Furthermore, good acceptability of beef patties containing 10% tempeh was observed compared to the 100% beef control, with better sensory properties regarding the perception of tenderness and juiciness [215]. This outcome coincides with the participants’ desire to choose more balanced and antioxidant-rich foods. However, it should be noted that food acceptability is also important if it is to be used routinely. While acceptability in populations that traditionally use tempeh may be high, in other countries where it is a little-known food, the aspect of sensorial perception should not be underestimated. Neophobia can be a decisive element in the acceptability of culturally new foods, such as tempeh, in some countries [222]. The acceptance of tempeh-like products in the Danish market could depend on the consumers’ predisposition to new foods, with a greater propensity by those who were already oriented towards plant-based alternatives, avoiding mimicking the meat-based foods characteristics [223]. Consequently, although it may be more difficult to include tempeh in the diet of populations with very different culinary traditions, the search for sustainable food options could lead to greater acceptance.
It has been proposed that tempeh may have a higher protein delivery efficiency
energy score compared to many foods of animal origin and this represents an
advantageous environmental aspect [224]. The most burdensome component on the
environmental impact of tempeh appears to be land use and eutrophication derived
from soy cultivation. Tempeh exerts a reduction in greenhouse gas (GHG)
production compared to pork, beef, chicken, fish, eggs, and milk, evaluated in
grams of proteins per kg of CO
Furthermore, transformation by fermentation can be an optimal system for recovering by-products from other food chains, which is the case for tempeh gembus since it is obtained from the okara of tofu or soy milk production. Tempeh gembus has shown beneficial effects on total, low-density lipoprotein (LDL) and high-density lipoprotein (HDL) cholesterol fractions in women with hyperlipidaemia [226]. The effect could be mediated by the high quantities of fibres and several in vitro studies have suggested further beneficial properties of tempeh gembus [227, 228, 229, 230].
Tempeh is an ancient Indonesian food, which despite it being widely consumed in East Asian and in some Western countries, data on its effects on humans remains limited. Furthermore, literature on this topic consists of a large percentage of articles, which are not presented in the English language from countries where tempeh is traditionally consumed. This aspect makes it difficult to disseminate such information. There is a need for randomized and controlled clinical trials with an adequate design and size that focus on the functional and nutraceutical effects of tempeh. Although tempeh studies mainly discuss isoflavones bioavailability, available trials have often limited statistical power. Furthermore, considering the heterogeneity of microorganisms used in its production, an adequate study of the microbial consortium in multi-strain pools could improve information regarding the functional properties of tempeh. Instead, the single-strain fermentation step process is widespread in the world and this could limit some benefits.
Finally, tempeh is a highly appreciated food with a high nutritional value and with a long history of use and consequent safety; thus, its commercial diffusion could increase, especially as a plant-based option to replace animal-based foods. This can improve health and environmental sustainability. Consistently, tempeh is an excellent candidate for the production of second-generation meat analogues. Global availability of animal protein is limited and does not allow adequate access to food for all populations. For this reason, the diffusion of a vegetable protein source such as tempeh would guarantee greater access to food and a lower ecological impact compared to the currently overexploited animal sources.
GR designed the research study, analyzed the information, and wrote and edited the manuscript. GR contributed to editorial changes in the manuscript. GR read and approved the final manuscript. GR has participated sufficiently in the work and agreed to be accountable for all aspects of the work.
Not applicable.
The author wishes to thank Sandra De Dominici for language revision assistance.
This research received no external funding.
The author declares no conflict of interest.
Publisher’s Note: IMR Press stays neutral with regard to jurisdictional claims in published maps and institutional affiliations.