† These authors contributed equally.
Background: Papillary thyroid cancer (PTC) is an endocrine malignancy whose incidence has increased rapidly worldwide. MAP17 (PDZKIP1) is a small protein related to tumor progression. The aim of this study was to investigate the role of MAP17 in PTC and the underlying molecular mechanism. Methods: Bioinformatics, Western blotting and immunohistochemistry were used to analyze the expression of MAP17 in PTC. The gene transcription was measured by qPCR. Cell viability was determined by CCK8 assay. Cell growth was measured by clonal formation assay. Cell apoptosis was measured by TUNEL. Wound healing assay and transwell assay were used to measure the mobility of cells. The expression of E-cadherin and N-cadherin was determined by immunofluorescence. The effect of MAP17 on tumor growth was determined in animal experiments. Results: The results showed that MAP17 was up-regulated in PTC, which significantly promoted the growth and motility of PTC cells, but inhibited cell apoptosis. Besides, overexpression of MAP17 accelerated cycloheximide (CHX, a protein synthesis inhibitor)-induced p53 degradation, while low expression of MAP17 slowed down CHX-induced p53 degradation, suggesting that MAP17 can regulate p53 stability. Notably, NUMB exhibited an opposite effect on P53 stability. Interestingly, p53 overexpression reversed the effects of MAP17 overexpression on cell viability, motility, and apoptosis, indicating that p53 was involved in the progression of PTC. In vivo studies have shown that tumor growth was positively correlated with MAP17 expression and negatively correlated with p53 expression. Conclusion: Our findings revealed that MAP17 exhibited carcinogenic effects through interacting with NUMB to reduce the stability of p53, demonstrating that MAP17 may serve as a potential prognostic biomarker for PTC treatment.
Papillary thyroid cancer (PTC) is an endocrine malignancy that occurs in thyroid follicular cells [1]. The incidence of PTC has increased rapidly worldwide over the last 3 decades. In the United States, the incidence of PTC increased 3.7-fold from 3.4 per 100000 to 12.5 per 100000 [2]. Most PTC patients have a good prognosis with a low mortality rate, but cervical lymph node metastasis is relatively common [3, 4]. Although great progress has been made for detection and treatment of PTC, the diagnostic accuracy still needs to be improved [5]. Therefore, it is urgently needed to develop alternative diagnostic therapeutic markers.
MAP17 (PDZKIP1) is an insoluble and non-glycosylated membrane-associated protein [6], which contains two transmembrane regions and a hydrophobic c-terminal encoding a postsynaptic density-95 (PSD-95), discs-large, zona occludens 1 (ZO-1) (PDZ) binding domain. MAP17 can interact with multiple proteins containing PDZ domains (Such as PDZK1) [7, 8]. MAP17 is overexpressed in various human cancers [9, 10], which is closely related to tumor progression [8, 9, 11], while MAP17 is rarely expressed in adenomas, benign tumors, and normal tissues, and a high proportion (50%–90%) of advanced or metastatic tumors always express high levels of MAP17, which is associated with a more dedifferentiated phenotype [8, 9, 10]. DNA methylation arrays showed that overexpression of MAP17 related to abnormal promoter hypomethylation may promote tumor growth in thyroid cancer. However, the specific role and underlying molecular mechanism of MAP17 in thyroid cancer are still unclear.
P53 is one of the most important tumor suppressors in cells, which has been found to occur deletion or mutation in more than 50% of human tumor cells. A large number of studies have shown that p53 also plays an important role in cell metabolism, especially glucose metabolism. In thyroid cancer, mutations of p53 occured in anaplastic carcinoma (AC), which is a rare but highly aggressive form of thyroid cancer [12]. NUMB can increase the stability of p53 and reduce p53 ubiquitination degradation [13, 14, 15]. Interestingly, MAP17 can interact with NUMB to inhibit its function [16]. However, whether MAP17 can regulate PTC tumor progression through interacting with NUMB/p53 remains to be investigated.
This study investigated the expression of MAP17 in PTC and the influence of abnormal expression of MAP17 on the progression of PTC. Our results showed that MAP17 was highly expressed in PTC, which was closely related to cell growth, motility and apoptosis. In vivo studies have confirmed that MAP17 overexpression significantly promotes tumor growth. Our findings suggest that MAP17 may serve as a potential target for PTC treatment.
A tool named UALCAN (http://ualcan.path.uab.edu/index.html) is generally used for TCGA data mining analysis to retrieve the expression of multiple genes in tumors as well as the relationship of the expression with prognosis [17]. In this study, the transcriptional expression of MAP17 (also known as PDZK1IP1) in PTC and normal thyroid tissues were analyzed using UALCAN, with a cut-off p value of 0.01.
GEPIA (http://gepia.cancer-pku.cn/index.html), developed by Peking University, is an interactive analysis website containing abundant RNA sequencing expression data from TCGA and GTEx. GEPIA has various specific roles, such as tumor/normal differential expression analysis, and analysis based on cancer type or pathological stage [18]. In this study, MAP17 (also known as PDZK1IP1) was analyzed by multi-gene comparison module. In addition, the single gene analysis module of GEPIA was used to explore the relationship between MAP17 expression and pathological stage.
A total of 50 PTC tissues and para-cancerous normal tissues from patients with PTC were collected during surgery procured from People’s Hospital, People’s Hospital of Hangzhou Medical College. The expression of MAP17 in PTC tissues and para-cancerous normal tissue was assessed by immunohistochemistry. In brief, the tissues were embedded in paraffin, and incubated with anti-MAP17 antibody (ab156014, 1:250, Abcam, Cambridge, UK). MAP17 staining was observed under a microscope. All analyses were conducted in triplicate.
PTC cell lines (HTh-7, KHM-5M, CAL62, KTC-1 and TPC-1) and normal human normal
thyroid HTori-3 cell line were purchased from the Cell Bank of the Shanghai
Chinese Academy of Sciences (Shanghai, China). Cells were cultured in
high-glucose DMEM (Hyclone; Thermo Fisher Scientific, Waltham, MA, USA)
containing 10% FBS and 1% antibiotic-antimycotic in an incubator at 37
The levels of MAP17, p53,
For overexpression, MAP17 was overexpressed using adenovirus system. Briefly,
constructs (MAP17-overexpressing (MAP17) and negative control (NC)) were inserted
into an adenovirus-packaging plasmid. After that, the above adenovirus were
packaged in HEK293A cells [19]. CAL62 and TPC-1 cells were seeded into 24-well
plates, achieving to a cell density of about 2
Cell viability or the effects of MAP17 on proliferation were determined using
CCK8 assay. CAL62 and TPC-1 cells at a density of about 4
After transfection, CAL62 and TPC-1 cells (400 cells/well) in the logarithmic
phase were seeded in 6-well plates and incubated at 37
Apoptosis was determined by TUNEL staining using In Situ Cell Death
Detection Kit (Roche). CAL62 and TPC-1 cells were inoculated into 24-well plates
at a density of about 2
CAL62 and TPC-1 cells were plated in 24-well plates and cultured until
monolayers formed. The monolayers were subjected to mitomycin C at 75
Invasion was determined by transwell assay. CAL62 and TPC-1 cells were
serum-starved for 24 h, and then inoculated into upper chambers coated with
Matrigel (BD Biosciences, Bedford, MA, USA) at a density of 2
The levels of E-cadherin and N-cadherin were detected by immunofluorescence staining. CAL62 and TPC-1 cells grown in 96 well plates were washed with PBS, fixed with 4% paraformaldehyde, and sealed with 5% BSA/PBS for 30 min. The cells were then incubated with primary antibodies of E-cadherin (1:200, #3195, Cell Signaling Technology, Danvers, MA, USA) and N-cadherin (1:200, #13116, Cell Signaling Technology, Danvers, MA, USA). Thereafter, the cells were stained with fluorescein isothiocyanate or Cy3 linked secondary antibody, and imaged by cellomics ArrayScan HCS reader (Thermo Science, Waltham, MA, USA).
Female BALB/c nude SPF mice (6 weeks of age, 18–22 g in weight) were provided
by Vital River (Beijing, China). Animals were kept at room temperature of 18–25
The expression of Ki67, MAP17 and p53 in tumor tissues was determined using
immunohistochemical staining as previously described [20]. In brief, tissue
sections were dewaxed with xylene and hydrated with different concentration
gradients of ethanol. The sections were then immersed in a pH 6.0 sodium citrate
buffer and boiled for 30 min. Sections were incubated at 37
Data are presented as means
We first analyzed the expression of MAP17 in PTC using GEPIA database and UALCAN database. The expression of MAP17 was increased in PTC (Fig. 1A,B), and the level of MAP17 increased over with lymph node metastasis (Fig. 1C). Besides, the expression of MAP17 in tall papillary thyroid carcinoma was the highest (Fig. 1D). In addition, tumor tissue samples and para-cancerous tissue samples from patients with PTC were collected during the surgery, and MAP17 expression was detected by PCR, immunohistochemistry and Western blotting. MAP17 expression was increased at both mRNA (Fig. 1E) and protein levels in tumor tissues compared with adjacent tissues (Fig. 1F–G). Overall, these evidences consistently indicated that the expression of MAP17 was increased in patients with PTC.
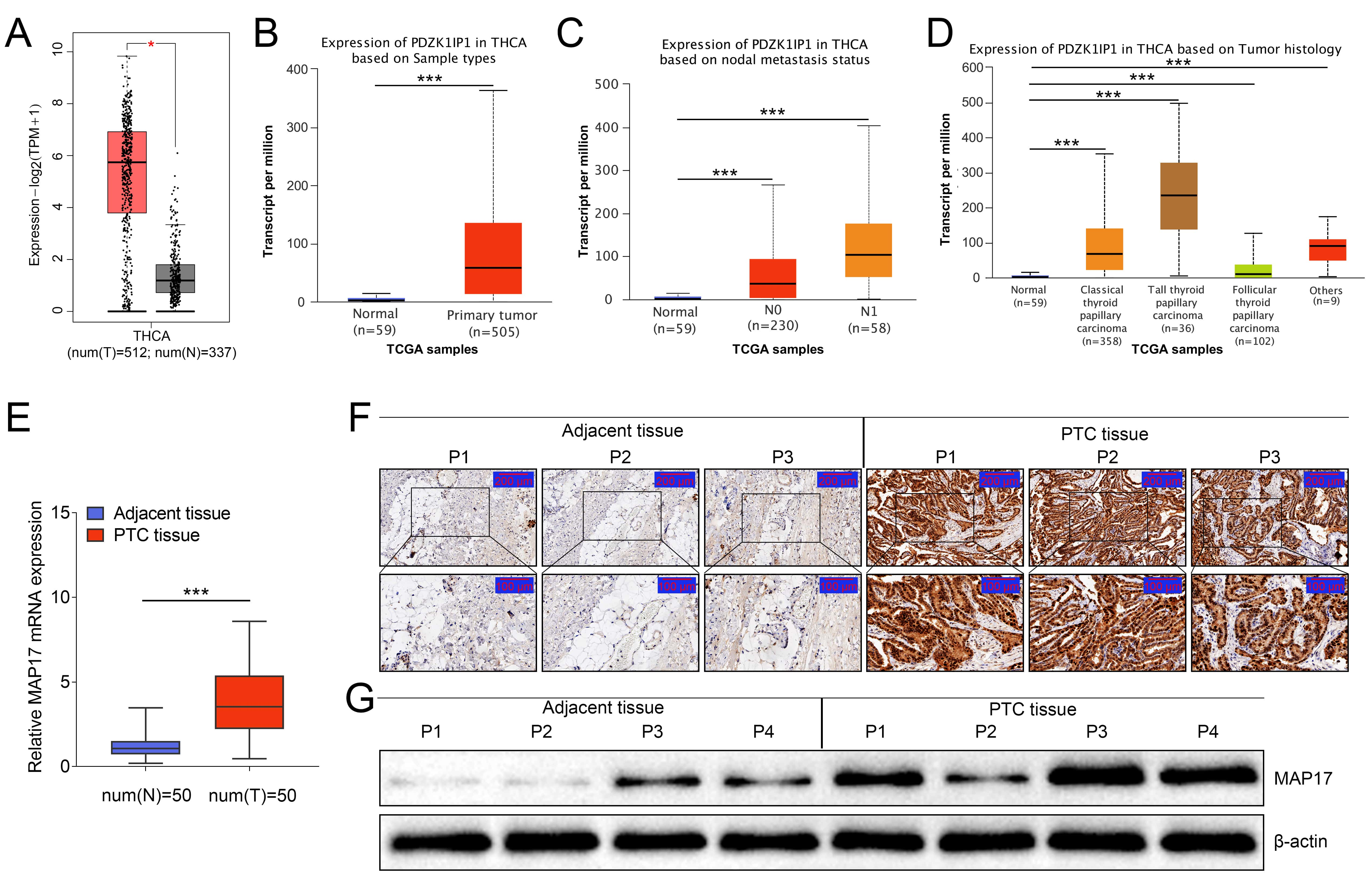
The expression of MAP17 was increased in patients with PTC.
(A–D) MAP17 expression was analyzed in GEPIA database and UALCAN database
(
We investigated the expression and role of MAP17 in PTC in vitro. The expression of MAP17 in Htori-3 cells and PTC cell lines (HTH-7, CAL62, KHM-5M, TPC-1 and KTC-1) was determined by Western blotting. As shown in Fig. 2A, MAP17 expression in PTC cell lines was significantly increased compared with that in Htori-3 cells, and CAL62 and TPC-1 cells were selected for further study. To further explore the effect of MAP17 on PTC cells, MAP17 was overexpressed or knocked down in CAL62 and TPC-1 cells, respectively. As shown in Fig. 2B, the levels of MAP17 in CAL62 and TPC-1 cells were significantly increased after the cells were infected with adenovirus overexpressing MAP17, while decreased after the cells were transfected with shRNA (shRNA#1 and shRNA#2), indicating that MAP17 had been successfully overexpressed or knocked down in this study. In addition, the effects of abnormal MAP17 expression on the growth and apoptosis of CAL62 and TPC-1 cells were determined by clone formation assay and TUNEL staining, respectively. As shown in Fig. 2, the overexpression of MAP17 significantly increased cell viability of PTC cells (Fig. 2C), and promoted the growth of PTC cells (Fig. 2D), as well as inhibited the apoptosis of PTC cells (Fig. 2E), while the low expression of MAP17 showed opposite effects. These results indicated that MAP17 promoted the growth of PTC cells and inhibit cell apoptosis in vitro.
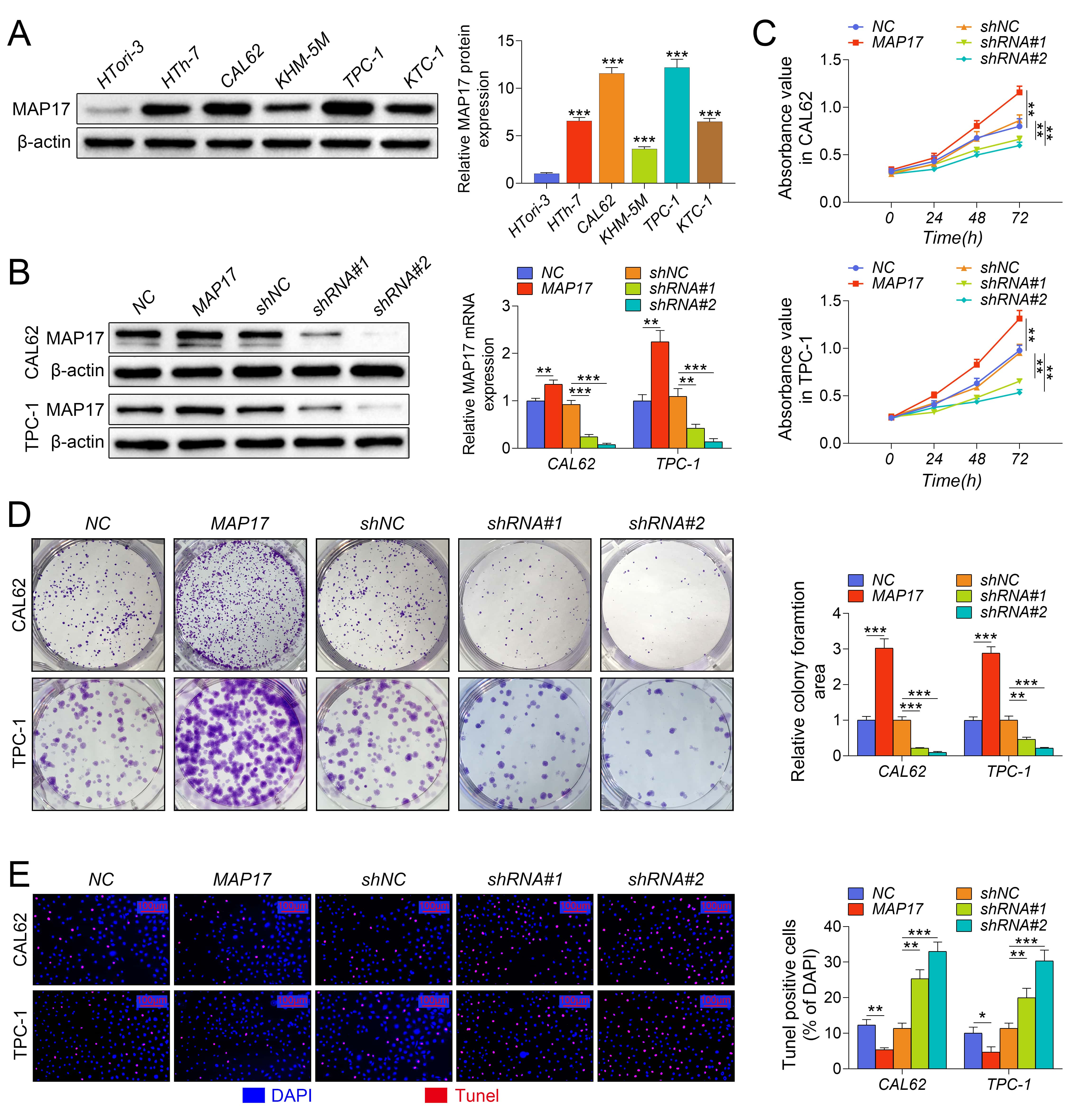
MAP17 promoted the growth of PTC cells and inhibited cell
apoptosis in vitro. (A) The expression of MAP17 in Htori-3
cells and PTC cell lines (HTH-7, CAL62, KHM-5M, TPC-1 and KTC-1) was detected by
western blotting (
In addition, we further investigated the effect of abnormal MAP17 expression on the motility of CAL62 and TPC-1 cells. As shown in Fig. 3A,B, the overexpression of MAP17 significantly promoted the invasion and migration of PTC cells, while the low expression of MAP17 inhibited the invasion and migration of PTC cells. Furthermore, immunofluorescence analysis showed that MAP17 overexpression significantly increased the level of N-cadherin, but decreased the level of E-cadherin. The low expression of MAP17 showed an opposite effect, indicating that MAP17 promoted the process of EMT (Fig. 3C). In summary, these results showed that MAP17 promoted the motility of PTC cells.
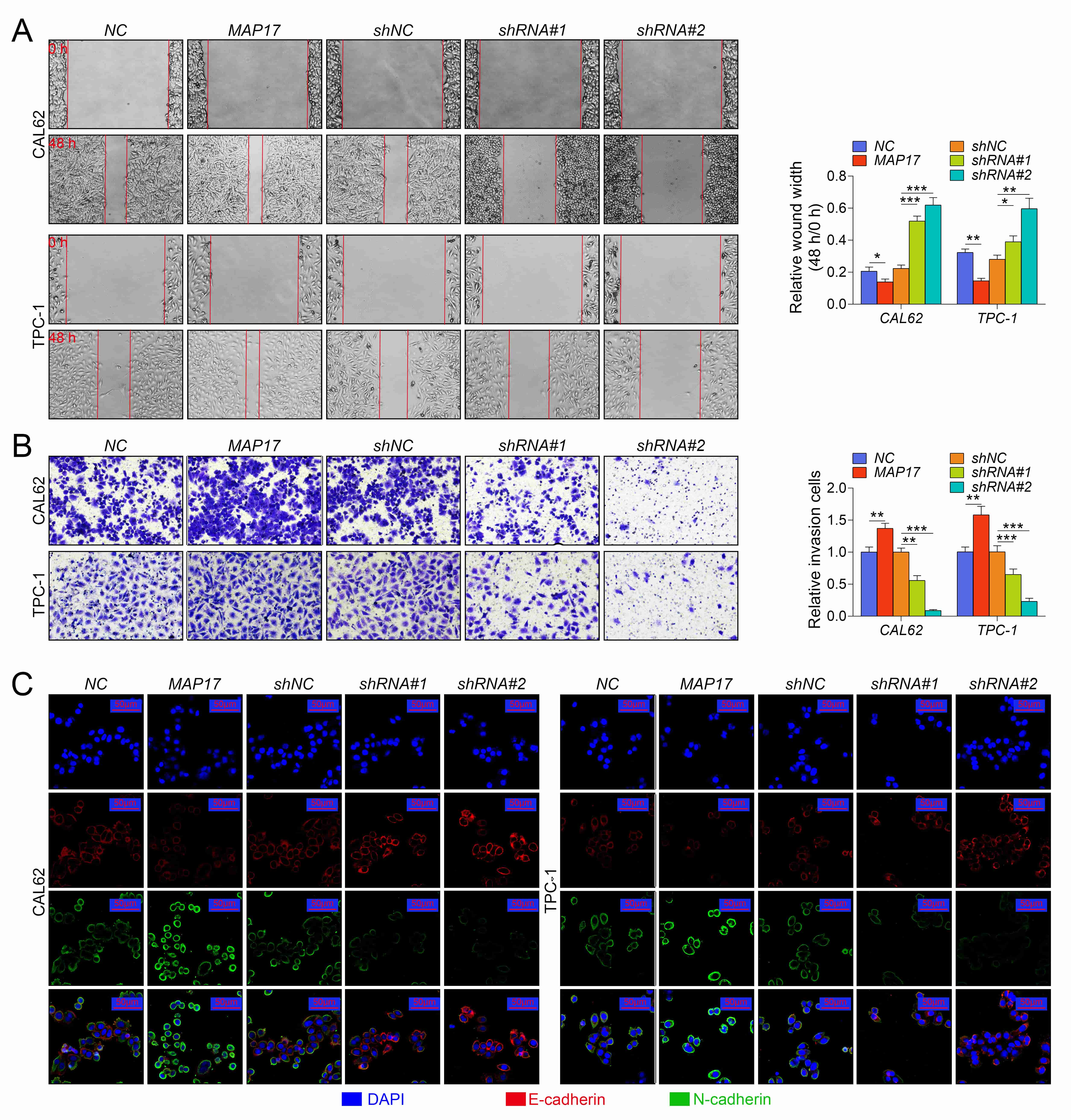
MAP17 promoted the migration, invasion and epithelial-mesenchymal
metastasis of PTC cells. CAL62 and TPC-1 cells were infected with adenovirus
containing MAP17, or transfected with shRNAs (shRNA#1 or shRNA#2). (A) Cell
migration was measured by wound healing assay. (
Studies have shown that NUMB could inhibit p53 ubiquitination, and enhance the stability of p53, and MAP17 could physically interact with NUMB. Therefore, this study investigated the effect of MAP17 on NUMB mediated p53 stabilization. As shown in the Fig. 4A–B, RT-qPCR showed that the abnormal expression of MAP17 had no significant effect on the transcription of p53, but significantly decreased the p53 expression in CAL62 and TPC-1 cells, while MAP17 knockdown significantly increased the protein level of p53 (Fig. 4A,B). After MAP17 was overexpressed or knocked down in TPC-1 cells, TPC-1 cells were subjected to the protein synthesis inhibitor cycloheximide (CHX) for 0, 0.5, 1 and 2 h, respectively. Western blotting showed that in the presence of CHX, the overexpression of MAP17 accelerated the degradation of p53, while the knockdown of MAP17 slowed the degradation of p53 (Fig. 4C). Furthermore, CAL62 or TPC-1 cells were infected with adenovirus overexpressing MAP17 or NUMB, either alone or in combination. The results showed that MAP17 overexpression significantly promoted p53 degradation in CAL62 or TPC-1 cells, while NUMB overexpression significantly inhibited p53 degradation in CAL62 or TPC-1 cells (Fig. 4D). Further study showed that p53 degradation was significantly slowed in CAL62 or TPC-1 cells after co-infection with adenovirus overexpressing MAP17 and NUMB. Notably, NUMB did not affect the expression of MAP17 (Fig. 4E). Taken together, these results suggested that MAP17 reversed NUMB-mediated p53 stabilization.
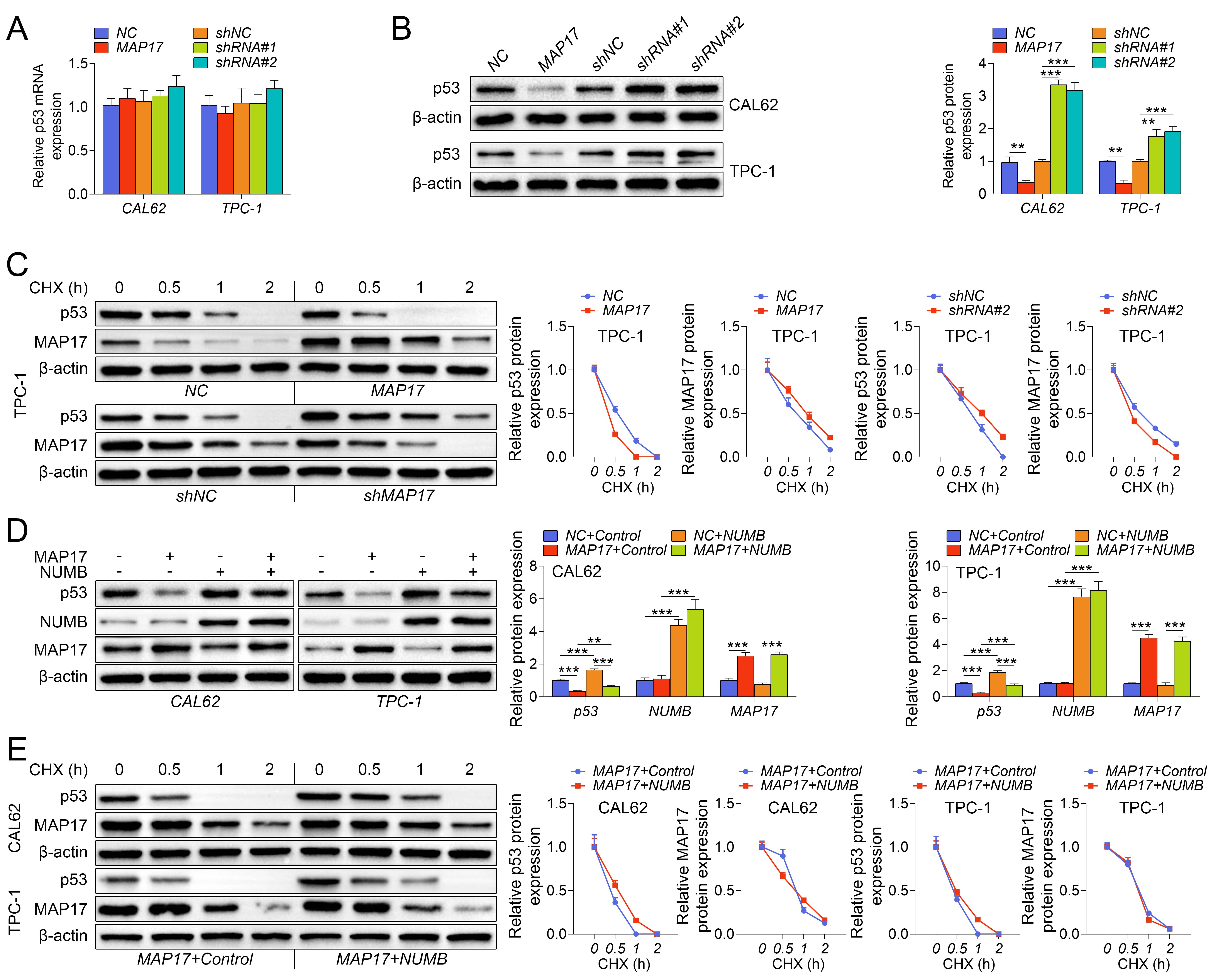
MAP17 reversed NUMB-mediated p53 stabilization. CAL62 and TPC-1
cells were infected with adenovirus containing MAP17, or transfected with shRNAs
(shRNA#1 or shRNA#2). (A) The expression of p53 in cells was detected by
RT-qPCR. (B) The expression of p53 in cells was detected by western blotting. (C)
TPC-1 cells were infected with adenovirus containing MAP17, or transfected with
shRNA#2, and then subjected to the protein synthesis inhibitor cycloheximide
(CHX) for 0, 0.5, 1 and 2 h, respectively. The expression of p53 and MAP17 in
cells was detected by western blotting. (D) CAL62 or TPC-1 cells were infected
with adenovirus overexpressing MAP17 or NUMB, either alone or in combination. The
expression of p53, NUMB and MAP17 in cells was detected by western blotting
(
Previous results in this study showed that MAP17 accelerated p53 degradation, thereby reducing p53 levels in cells. Therefore, to investigate whether p53 is involved in the progression of the tumor in this study, TPC-1 cells were infected with adenovirus containing MAP17 or p53 to overexpress MAP17 or p53, respectively. Cell viability was examined by CCK8 assay, while cell growth was measured by clone formation assay. As shown in Fig. 5A and B, MAP17-induced low expression of p53, which significantly improved the viability of TPC-1 cells, and promoted cell growth, but p53 overexpression exhibited the opposite effect. However, the co-transfection of MAP17 and p53 significantly reversed the promotional effect of MAP17 overexpression on the growth of TPC-1 cells. Similarly, MAP17-induced low expression of p53 significantly inhibited apoptosis in TPC-1 cells, while p53 overexpression showed the opposite effect. However, the co-transfection of MAP17 and p53 significantly reversed the inhibitory effect of MAP17 overexpression on the apoptosis of TPC-1 cells (Fig. 5C). Furthermore, MAP17-induced low expression of p53 significantly promoted the mobility of TPC-1 cells, while p53 overexpression showed the opposite effect. However, the co-transfection of MAP17 and p53 significantly reversed the promotional effect of MAP17 overexpression on the mobility of TPC-1 cells (Fig. 5D–E). Taken together, these results suggested that MAP17 promoted the growth and metastasis of PTC cells through degrading p53.
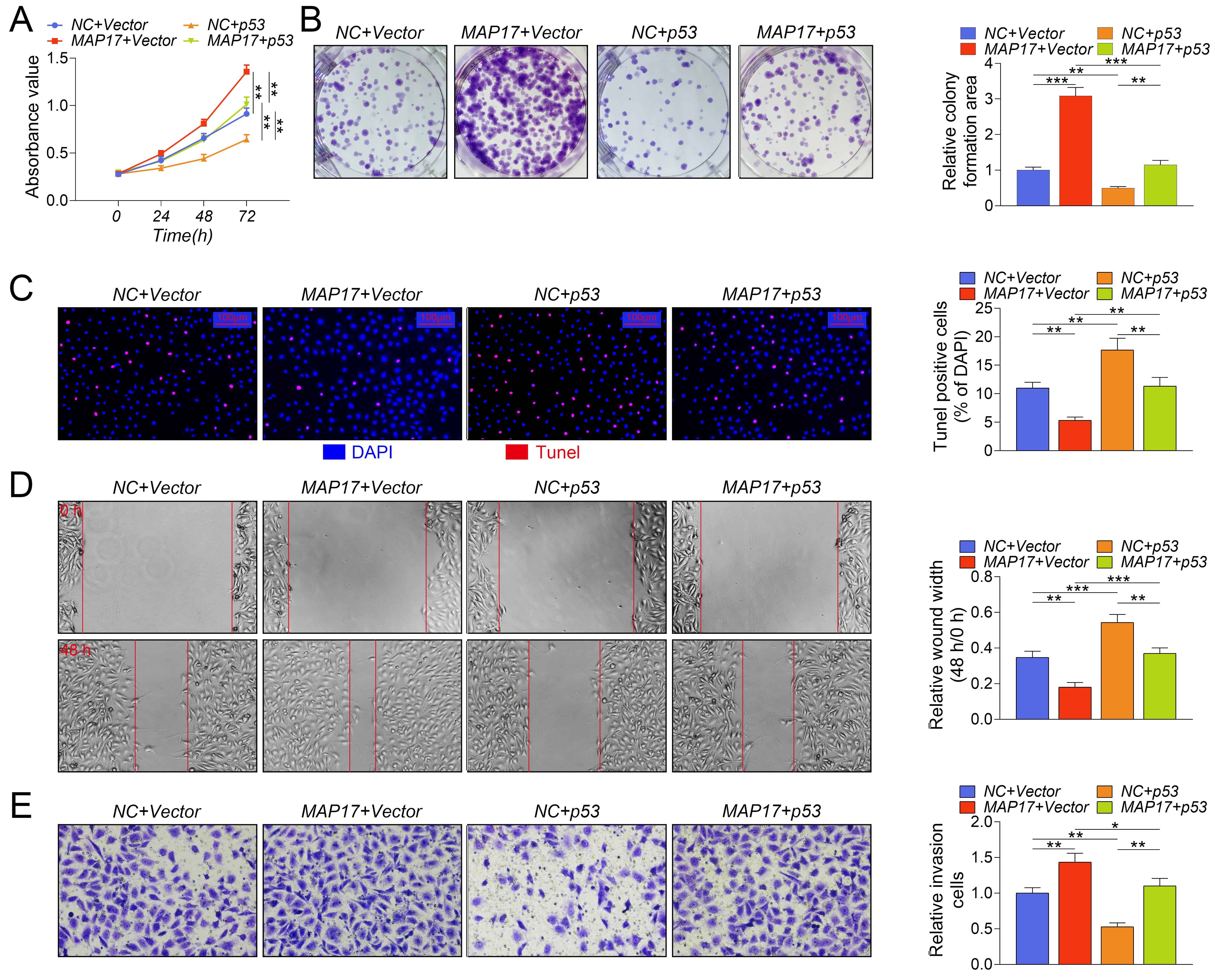
MAP17 promoted the growth and metastasis of PTC cells by p53.
TPC-1 cells were infected with adenovirus containing MAP17 or p53 to overexpress
MAP17 or p53, respectively. (A) Cell viability was examined by CCK8 assay. (B)
Cell growth was measured by clone formation assay. (C) Cell apoptosis was
measured by TUNEL staining. (D) Cell migration was measured by wound healing
assay. (E) Cell invasion was measured by transwell assay (
TPC-1 cells over-expressing and low-expressing MAP17 were injected subcutaneously into BALB/c nude mice, and tumor volume and weight were measured. As shown in Fig. 6A–C, MAP17 overexpression significantly promoted tumor growth, while MAP17 knockdown significantly inhibited tumor growth. Besides, immunohistochemistry showed that MAP17 overexpression significantly promoted the expression of Ki67, but inhibited the expression of p53 in tumor tissues. On the contrary, MAP17 knockdown got an opposite result (Fig. 6D). Taken together, these results suggested that knockdown of MAP17 inhibited tumor growth in vivo.
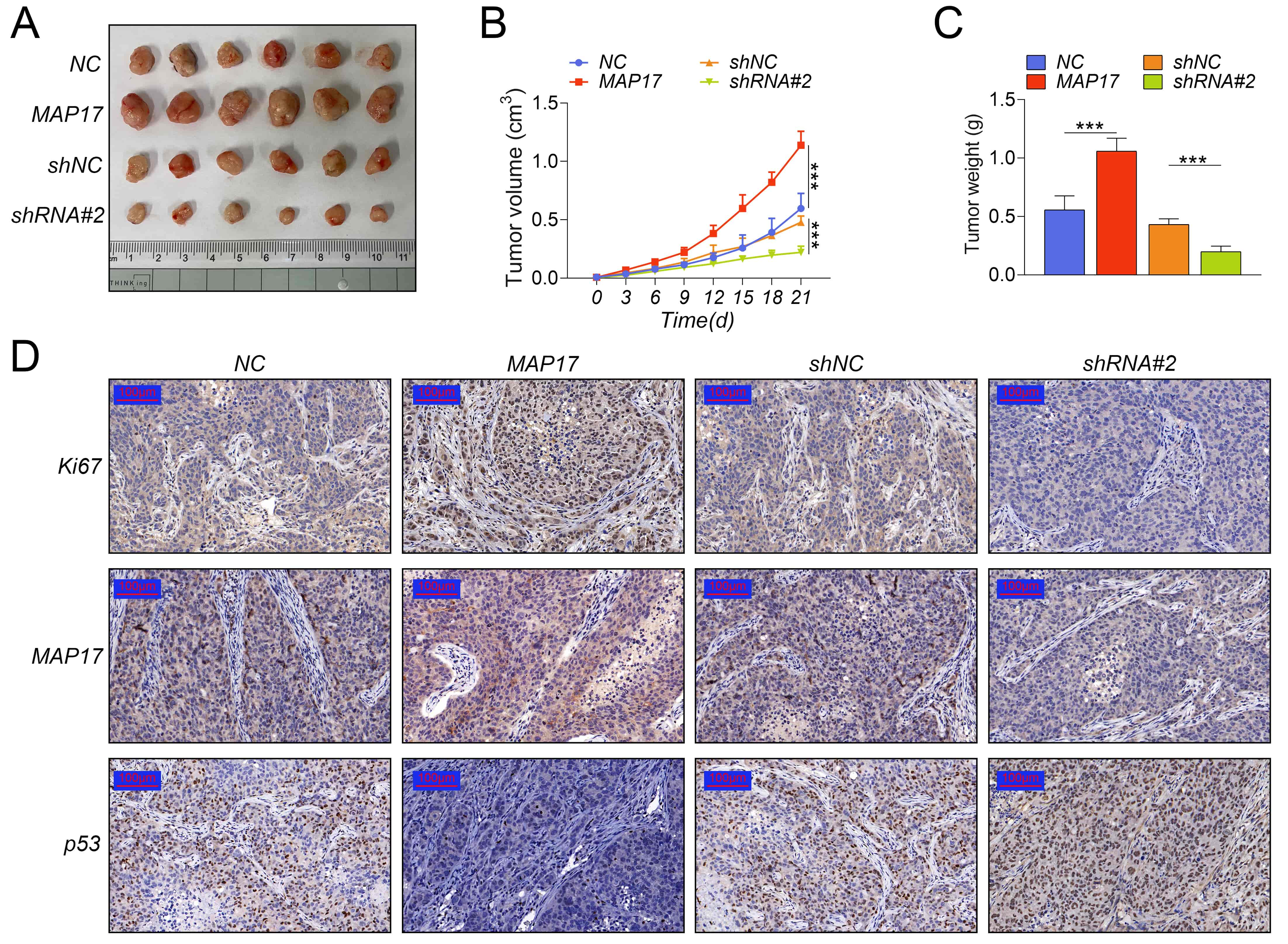
Knockdown of MAP17 inhibited tumor growth in
vivo. TPC-1 cells over-expressing and low-expressing MAP17 were
injected subcutaneously into BALB/c nude mice. Tumor bearing mice were randomly
divided into four groups (10 mice per group): NC group, MAP17 group, shNC group
and shRNA#2 group. (A) Tumors. (B) Tumor volume. (C) Tumor weight. (D) The
expression of Ki67, MAP17 and p53 were measured by immunohistochemistry
(
In recent years, the incidence of PTC has increased globally, but the mortality rate is decreasing [21, 22]. Therefore, novel molecular markers are essential for risk stratification and personalized management of PTC [23]. Previous studies have revealed multiple potential PTC markers, such as miRNAs (miR-136, miR-21, and miR-127) [19], lncRNA SLC26A4-AS1 [24], and Lactate Dehydrogenase A [25]. Fortunately, in this study, we identified a novel potential molecular marker for PTC treatment, i.e., MAP17. Bioinformatics analysis demonstrated that MAP17 was highly expressed in PTC, which was further confirmed by in vitro and in vivo studies. Specifically, the high expression of MAP17 was associated with excessive proliferation and reduced apoptosis in PTC cells, as well as tumor growth. Consistently, the carcinogenic effects of MAP17 have also been demonstrated in non-small cell lung cancer and breast cancer [26, 27]. Collectively, it suggested that MAP17 may serve as a potential prognostic marker for PTC.
MAP17 is a small protein related to tumor progression that regulates inflammation and tumor progression [28]. Studies have shown that MAP17 has potential prognostic roles in tumors, such as lung cancer, especially in lung adenocarcinoma [28]. Besides, MAP17 overexpression enhanced stem cell characterization of cells, and promoted microsphere formation and stem cell-related gene transcription by interacting with NUNB [16]. However, MAP17 is still poorly studied in PTC. Preciously, Sandra Rodríguez-Rodero et al. [29] found that abnormal overexpression of MAP17 promoter associated with hypomethylation may promote tumor growth in thyroid cancer. As supplementary, in this study, it was found that the level of MAP17 was significantly up-regulated in PTC, which was further verified by the analysis of the GEPIA database and the UALCAN database. Besides, we also found that overexpression of MAP17 significantly promoted the progression of PTC. Indeed, it was considered that the carcinogenic effect of MAP17 was achieved mainly by activating the Notch pathway [30], which changed the phenotype of cancer stem cells [16]. Inconsistently, in our study, it was found that MAP17 exhibited carcinogenic effects through interacting with NUMB to reduce the stability of p53.
MAP17 was found to be physically combined with NUMB, leading to a mislocalization of NUMB, thereby activating the Notch pathway [16]. Interestingly, NUMB was found to bind to and stabilize p53, thereby exhibiting a suppressive effect on tumor [13]. However, methylation can uncouple NUMB from p53, thereby increasing p53 ubiquitination and degradation. In this study, we found that abnormal expression of MAP17 significantly reduced the protein level of p53, but has no effect on the transcription of p53 in vitro. To further clarify the mechanism of action between MAP17 and p53, cells were subjected to synthesis inhibitor CHX for various hours. After CHX treatment, the protein levels of p53 and MAP17 in TPC-1 cells were significantly reduced, indicating that CHX successfully inhibited the protein synthesis of p53 and MAP17 in TPC-1 cells. Interestingly, overexpression of MAP17 enhanced the inhibitory effect of CHX on p53 protein synthesis, while MAP17 knockdown counteracted the inhibitory effect of CHX on p53 protein synthesis. Furthermore, we found that overexpression of NUMB reversed the reduction of p53 protein levels mediated by MAP17. As expected, we found that compared with overexpression of MAP17 alone, simultaneous overexpression of MAP17 and NUMB not only maintained the stability of p53, but NUMB also bound to a part of MAP17, thereby reversed the inhibitory effect of MAP17 on p53 synthesis. In addition, our study further confirmed that p53 is involved in the carcinogenic effects of MAP17. It was found that the reduction of p53 induced by MAP17 significantly promoted cell growth and motility, and inhibited cell apoptosis, which was significantly reversed by forced overexpression of p53, indicating that p53 is involved in the carcinogenic effects of MAP17.
In this study, the expression of MAP17 in PTC was measured in vitro and in vivo, and the effects of MAP17 on PTC were measured from in vitro studies and animal experiments. The results showed that MAP17 expression was increased in PTC, which is associated with poor prognosis of patients with PTC, cell growth and motility, as well as tumor growth. MAP17 knockdown inhibited the proliferation and motility of PTC cells and promoted apoptosis. Taken together, our results suggested that MAP17 exhibited carcinogenic effects by interacting with NUMB to reduce the stability of p53.
KY and HL designed the study, supervised the data collection, YC and YX analyzed the data, interpreted the data, ZT and QY prepare the manuscript for publication and reviewed the draft of the manuscript. All authors have read and approved the manuscript.
The study was approved by the Medical Ethics Committee of Zhejiang Provincial People’s Hospital (Approval No. 2016QT160). Animal experiment was approved by the Laboratory Animal Welfare and Ethics Committee of Zhejiang University (Approval No. ZJU20190092).
Not applicable.
This work was supported by The General Project of the Medical and Health of Zhejiang Province (Grant Number: 2021KY493).
The authors declare no conflict of interest.