- Academic Editor
Breast cancer (BC) is the second most common malignancy in the world. Numerous studies have demonstrated the association between human leukocyte antigen (HLA) and cancer. The occurrence and development of BC are closely linked to genetic factors. Human leukocyte antigens G and E (HLA-G and HLA-E) are non-classical major histocompatibility complex (MHC) class I molecules. These molecules play an important role in immune surveillance by inhibiting the cytotoxic and natural killer T cells responsible for immune escape. The expression of HLA-G and HLA-E has been associated with several diseases, including tumors. The HLA system plays a key role in the escape of tumor cells from immune surveillance. This review aims to determine the correlation between BC susceptibility and HLA markers specific HLA alleles such as HLA-B07, HLA-DRB111, HLA-DRB113, and HLA-DRB115 are associated with an increased risk of developing BC. Furthermore, HLA-G mutations have been attributed to an elevated likelihood of metastasis in BC patients. Understanding the complex associations between the HLA system and BC development is critical for developing novel cancer prevention, detection, and treatment strategies. This review emphasizes the importance of analyzing HLA polymorphisms in the management of BC patients, as well as the urgent need for further research in this area.
Breast cancer (BC) is a highly intricate and varied ailment that presents an ongoing and pressing challenge to the global public health community. This disease has resulted in a substantial rise in mortality rates among women, making it the most widespread form of cancer affecting women on a global scale. The etiology of the disease can be attributed to the unregulated proliferation of abnormal cells inside the breast tissue, leading to the formation of malignant tumors [1, 2, 3].
While the underlying cause of BC remains poorly understood, extensive research has identified several known risk factors contributing to its occurrence. One prominent risk factor is age, with a strong correlation between BC incidence and age, particularly among women over the age of 50 [4, 5].
Family history and genetic variables also play a significant role in cancer development. Certain gene mutations, such as BRCA1 and BRCA2, are associated with an increased susceptibility to BC. However, it’s worth noting that most BC cases occur in women with no family history of the disease [6].
Moreover, the use of oral contraceptives and hormone replacement therapy (HRT) exposes women to estrogen, thereby elevating their likelihood of developing BC. The risk of BC has been proven to rise with prolonged use of HRT, especially when estrogen and progesterone are combined [7]. Lifestyle-related factors, such as heavy alcohol consumption, inactivity, and a high-fat diet, have also been linked to an increased risk of BC. Additionally, obesity, particularly after menopause, is another known risk factor [4].
To effectively combat BC, further research is required to better comprehend its underlying mechanisms and the interplay of various risk factors. Implementing comprehensive prevention and screening programs, as well as advancing treatment options, are critical in the ongoing battle against this devastating disease.
The human leukocyte antigen (HLA) super-locus is on chromosome 6p21 and is home to the human major histocompatibility complex (MHC) genes. More than 250 annotated genes and pseudogenes are expressed in this genomic complexity; these genes are often divided into three regions called classes II, III, and I. Some of the MHC genes are clustered together in haplotypes that code for proteins involved in heat shock, the complement cascade, cytokine signaling, and the regulation of cell development, differentiation, and apoptosis, as well as the presentation of cellular and extracellular antigens to circulating T cells [8]. It is well acknowledged that HLA genes are linked to certain diseases. Evidence linking HLA genes to over 40 diseases has been uncovered via many population-based studies. HLAs are found to vary widely among populations [9].
The two HLA class I and II protein complexes form bonds with peptides and present them to T cell receptors. This is how the immune response begins. B-lymphocytes produce specific antibodies, cytokines, and cytotoxic lymphocytes [10].
In addition, traditionally, heritability has been estimated using family-based methods such as twin studies. Studies using sibling linkage analysis revealed one of the first reproducible associations in IBD—in fact, in complex inherited diseases in general—the NOD2 polymorphisms [11].
Indeed, spondyloarthritis (SpA) is an autoimmune disease with a significant family clustering pattern. Therefore, family studies are an effective method of uncovering the genetic underpinnings of SpA. First, they have allowed researchers to determine the disease’s polygenic nature and the relative impact of genetic and environmental components [11].
A recent meta-analysis showed differences in the frequencies of HLA haplotypes in the populations analyzed. Studies on the frequency of different genetic loci and anthropological traits clearly illustrate the geographical and genetic barrier between Europeans and Asians in northwest China [12].
Frequencies of HLA alleles and haplotypes are essential for the study of HLA-associated diseases; therefore, it is crucial to maintain accurate population-specific data for this purpose [13].
Recent years have seen a surge in research on the potential of selenium-rich functional foods to fight cancer. Daily dosages of 100 to 200 g of selenium may suppress genetic damage and the development of cancer in humans; however, clinical trials are equivocal and the anti-cancer mechanisms of selenium are not entirely understood [14].
The study of hydrophobic interactions of proteins generated by various dietary and/or environmental variables appears to be important in a number of diseases, such as cardiovascular disease, neurological dysfunction, and degenerative diseases such as cancer [15]. The body’s many systems rely on the trace element selenium to function normally, and this is especially true of the immunological, endocrine, metabolic, and cellular systems. This component has recently been recognized as a very promising treatment alternative for a wide range of diseases [16].
Recent studies have shown a connection between the immune system and the different polymorphisms linked with the human leukocyte antigen (HLA) system and the incidence and treatment of cancer [16]. The HLA system, also referred to as MHC, is integral to the immune response and intricately linked to the emergence of cancer. HLA molecules are cell surface proteins that help T cells, a subtype of immune cells, present antigens, which are small protein fragments derived from infections or aberrant cells. The immune system can detect and destroy cancer cells owing to this critical antigen presentation pathway [17].
The diversity of HLA molecules arises from significant polymorphisms, leading to genetic variations among individuals. This diversity enables HLA molecules to present a wide range of antigens, facilitating a robust defense against various pathogens, including cancer cells. However, specific genetic variants within the HLA genes may influence the likelihood of developing cancer [17]. While some HLA alleles have been associated with a decreased risk, others have been linked to an increased risk of certain cancer types. The complex pathways through which HLA molecules impact cancer development involve multiple factors. These include the identification and destruction of cancer cells by T cells, the activation of immune cells, and the production of cytokines—signaling molecules that regulate immunological responses. In essence, these various processes intricately mediate the interaction between HLA molecules and cancer development [18].
Recent research has highlighted the potential role of HLA polymorphisms in BC. Thus, the objective of this review is to explore the association between BC and HLA polymorphism, shedding light on its implications and significance in this particular cancer type.
BC remains a formidable and prevalent form of cancer among women globally, despite the existence of numerous benign lesions [19]. BC is the most common cancer in women worldwide, affecting around 2.1 million women every year. It is also the largest cause of cancer-related deaths in females. According to GLOBOCAN 2020, there will be 19.3 million new instances of cancer detected in 2020, with about 10.0 million deaths attributable to the disease. GLOBOCAN predicts that the number of cancer cases will increase to 28.4 million in 2040. Worldwide, female BC has surpassed lung cancer as the most common cancer (11.7%) [20].
While BC rates are often higher in more developed countries, there has been a significant increase in occurrence in practically every region of the world, with BC incidence rates varying greatly by geographic region [20]. According to statistics, BC is the most commonly diagnosed type of cancer in women. BC accounts for 24.2% of all cancer cases in women worldwide, with approximately one out of every four new cancer cases reported. This prevalence is found in approximately 154 of the 185 nations studied in the study. Northern America, Northern and Western Europe, New Zealand, and Australia have the greatest total incidence rates. In contrast, the majority of African and Asian countries have the lowest incidence rates. It should be noted that BC is the most common malignant tumor in Asian women [19] and exhibits a steep upward trend [21] (this may be due to the availability of screening tests). In 2012, 639,824 instances of BC were reported in the aforementioned countries. China recorded the most instances, with 187,213, followed by India, which reported 144,937 cases. Japan came in third with 55,710 cases, Indonesia followed in fourth with 48,998 cases, and Pakistan came in fifth with 34,038 incidents. Surprisingly, these five Asian countries collectively suffer a large burden, accounting for 73% (470,896 out of 639,824) of BC cases [20].
BC is a major cause of morbidity and mortality globally [22]. It accounts for approximately 55.6% of all cancer cases in poor nations and leads to 62.1% of cancer-related deaths [23]. It is the main cause of cancer-related death among women in developing nations, accounting for 14.3% of the total 324,000 fatalities [24]. On the other hand, BC is the second highest cause of death in women in more developed nations, after lung cancer, accounting for 15.4% of fatalities (198,000) and ranking as the fifth leading cause of death in the world, accounting for 522,000 deaths each year [24]. BC has a varying impact across various regions worldwide. The highest standardized death rate in Asia is 25.2 per 100,000 people, with Armenia, Lebanon, Jordan, and Syria coming in at 24.2/100,000, 24/100,000, 21.8/100,000, and 21.5/100,000, respectively [20].
Several factors, as previously noted, have been identified as potential contributors to the development of BC. These factors include advanced age, use of HRT after menopause, nulliparity (never having given birth), marital status (such as being unmarried or widowed), a family history of BC, a diet consisting of a low intake of vegetables and fruits, alcohol consumption, smoking, late onset of menopause, older age at menarche, delayed age of first pregnancy, physical inactivity, and postmenopausal obesity. These risk factors have been linked with an increased risk of BC, highlighting the significance of comprehending and addressing them for successful prevention and early detection efforts [25, 26, 27, 28]. Higher socioeconomic status (SES) has also been associated with an increased incidence of BC, both locally and individually [29]. Obesity and weight gain are yet another important risk factor for the development of BC. Several studies have investigated the correlation between BC and obesity, but the results have been inconsistent. According to some researchers, having a Body Masse Index (BMI) of more than 30 may increase the risk of BC in both premenopausal and postmenopausal women [30]. However, there are opposing viewpoints; there are comments indicating obesity may reduce the risk of BC during the premenopausal period but raise the risk after menopause. Women who have early menarche or menopause at a young age have a shorter exposure to estrogen hormones than those who have later menopause or early menarche [31]. These variables add to the complexity of understanding BC risk and highlight the significance of additional studies to clarify the relationship between these variables.
Nelson et al. [32] and Anderson et al. [33] identified a correlation between the age of the first full-term birth and the risk of BC. Women who have never had children, had a low number of pregnancies, and had their first pregnancy at a later age have an increased likelihood of developing BC than those who had their first delivery at a younger age or have had multiple pregnancies. In addition, Lee and colleagues reported an increased risk of BC by 3% for every year of childbearing delay [34]. Breastfeeding, on the other hand, is a modifiable risk factor, as mothers who do not breastfeed their children have an increased likelihood of developing BC than those who do. Additionally, the duration of breastfeeding is directly associated with the level of protection against BC, but the particular extent of its protective action remains unknown due to a lack of research on the subject.
On top of the above, BC development also shows connections to genetic factors, particularly mutations in the BRCA1 and BRCA2 genes. Individuals with a BRCA1 mutation were identified as having a higher lifetime likelihood of developing BC (60–70%) than BRCA2 mutation carriers (45–55%). Meanwhile, mutations in the TP53 gene can result in the early development of aggressive BC that usually identified in women between the ages of 20 and 30, with a median age of 25. Another significant mutation is identified in the phosphatase and tensin homolog (PTEN) gene, which is associated with a variety of illnesses such as Cowden syndrome and has a potential lifetime risk of developing BC of 50–85%. Similarly, the STK11 gene mutation is associated with Peutz-Jeghers syndrome, which carries a lifetime risk of up to 50% for BC development [35].
On the other hand, a CDH-1 gene germline mutation is associated with a 50% lifetime likelihood of developing BC. Although environmental factors or lifestyle choices may alter the likelihood of developing BC, mutations in genes like CHEK2, ATM, PALB2, and BRIP have a low to moderate penetrance. Also, mutations in the RAD51 gene and its related genes, RAD51C and RAD51D, as well as in BARD1, XRCC2, and the MRN complex (MRE11, RAD50, and NBS1), have been linked to an increased risk of BC, though the exact penetrance and prevalence of these mutations are still unknown. Of note, obesity is linked to BC because it causes women to produce more extra-glandular estrogen. BC can be triggered by the metabolic products of estrogen and hyperinsulinemia [36, 37].
In humans, the major histocompatibility complex (MHC) is located on the short
arm of chromosome 6, comprising an estimated 4
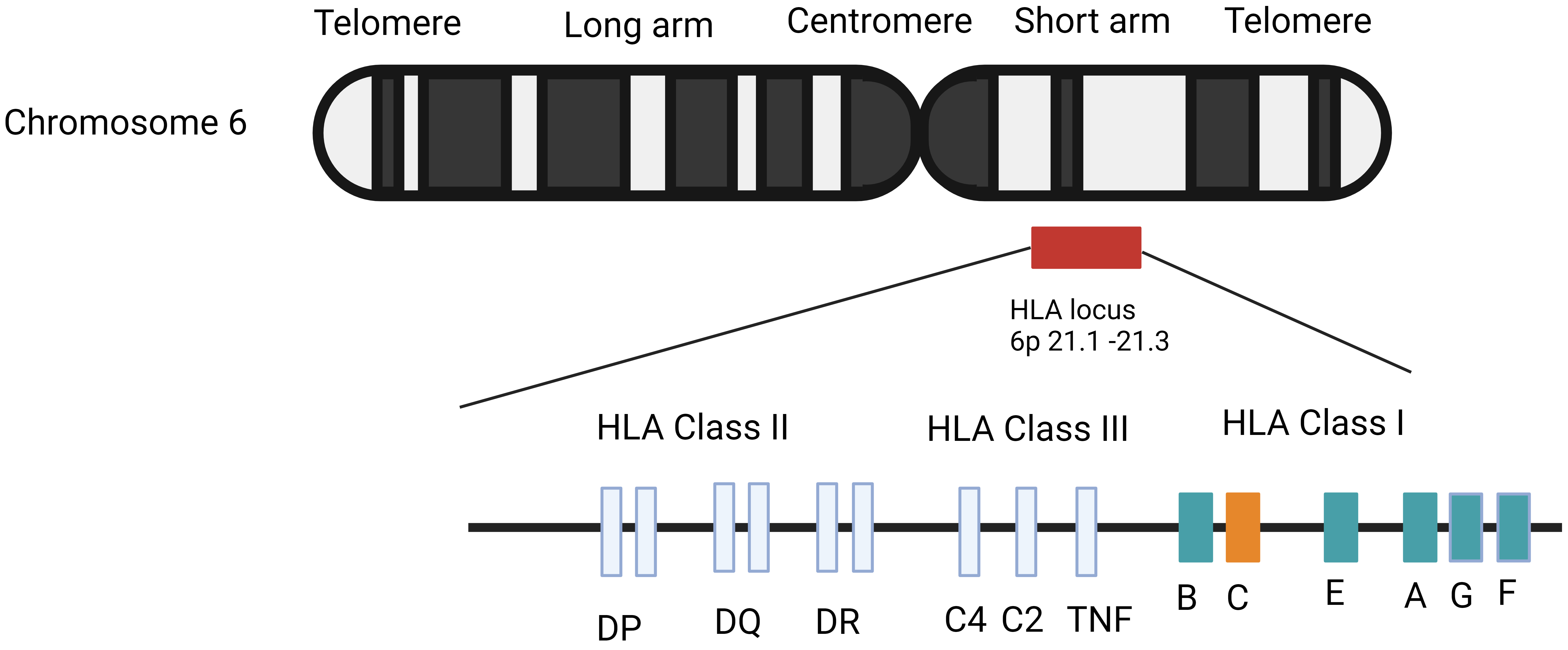
Genetic structure of human leukocyte antigen (HLA) genes. Adapted from Vollmers et al. [40] and Medhasi et al. [41].
The MHC region is further subdivided into three sub-regions known as classes I,
II, and III. MHC class I has six distinct genes, three of which are categorized
as major and three as minor. HLA-A, HLA-B, and HLA-C
are major class I genes, while HLA-E and HLA-F are minor class
I genes. Moving on to MHC class II, the HLA gene encodes three major
proteins as well as two minor proteins. In MHC class II, the HLA-DPA1
gene encodes the
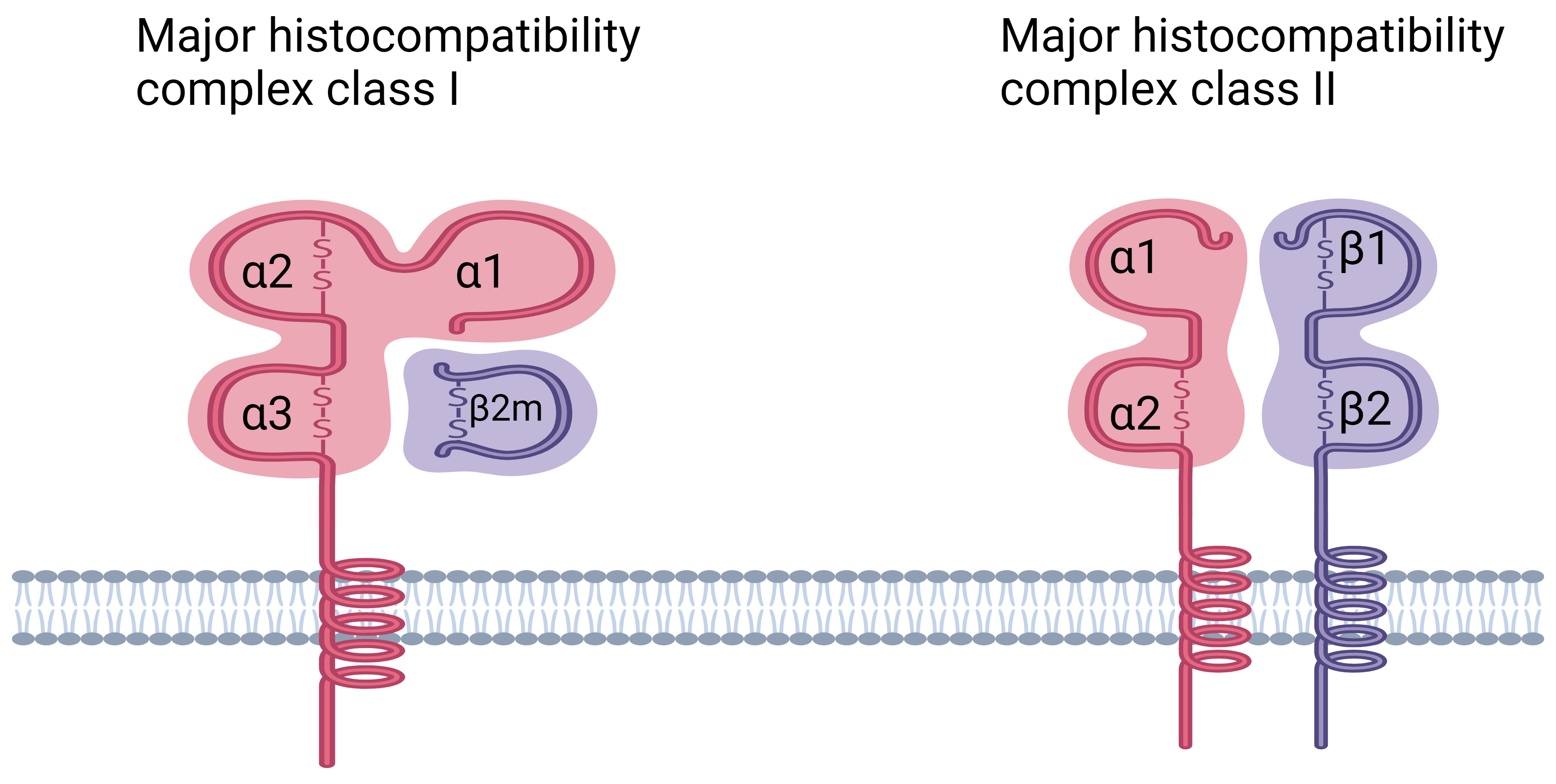
Structure of HLA class I and class II molecules. HLA class I molecules are composed of a
polymorphic heavy chain (
MHC class I molecules are essential for presenting peptides derived from cellular proteins. These peptides are normally derived from autologous proteins found in healthy cells, which renders them resistant to identification by CD8+ T lymphocytes. When cells express mutant sequences (as seen in malignancies), microbial genes (as seen in viral infections), or foreign polymorphic genes (as shown in transplants), “non-self” antigenic peptides are incorporated into the peptidome. As CD8+ T cells can recognize and respond to “non-self” antigenic peptides, they can identify and eradicate these abnormal cells [48].
MHC class I molecules are composed of two heterodimeric polypeptide chains, the
Thymic epithelial cells and peripheral antigen-presenting cells both express MHC
class II molecules on their cell surfaces. The
The adaptive immune system depends on antigen presentation by the host cell, which involves processing of the antigen inside the cell and binding to HLA molecules on the outside of the cell [52]. Exposure to viral infections has been widely considered a crucial factor leading to the broad diversity of HLA alleles observed in humans, which confers a protective advantage against viruses. Notably, HLA-G protein levels have been found to be adversely linked to poor clinical outcomes and are often discovered in a variety of human malignancies, indicating their role in disease development [53].
HLA-G plays a crucial role in various physiological and pathological processes,
encompassing reproduction, organ transplantation, autoimmunity, infection, and a
diverse array of infectious and malignant diseases. It is essential to highlight
that carcinogenesis cannot be attributed solely to human papillomavirus (HPV)
infection. A minority of HPV infections result in high-grade lesions and cervical
cancer, implying intricate host-virus interactions. HLA-G has emerged as a
potential therapeutic target and prognostic biomarker for a wide spectrum of
cancers and viral infections due to its extraordinary immunoinhibitory
capabilities. Its role in immune response modulation makes it an important
subject of research in these areas [54]. Immunosuppressive drugs and inflammatory
cytokines, such as IFN-
HLA-C overexpression in colorectal cancer cell lines has also been demonstrated to impair cell viability, confirming HLA-C’s functional importance in colorectal cancer. Researchers used ribonucleic acid (RNA) sequencing to compare HLA-C-overexpressing colorectal cancer cells to non-cancerous colorectal cells to acquire a better understanding of the underlying mechanisms associated with HLA-C in the development of colorectal cancer. Pathway analysis demonstrated that numerous cancer-related signaling pathways, including JAK/STAT, ErbB, and Hedgehog, were strongly enriched in the down-regulated genes observed in HLA-C overexpressing colorectal cancer cells. These findings shed light on the potential function of HLA-C in altering critical signaling pathways involved in colorectal cancer development [57].
Human leukocyte antigen-G (HLA-G) expression has been found to be abnormally high in many cancers. This is linked to tumor immune evasion, metastasis, and a poor prognosis. Indeed, E Eskandari-Nasab et al. 2013 [58] found that the homozygous 14-bp deletion genotype of HLA-G was more common in BC patients than in the control group, suggesting that the 14-bp insertion/deletion polymorphism in the HLA-G gene could be a genetic risk factor for breast carcinoma susceptibility [58]. Furthermore, based on HLA-G expression in the tissues, an untranslated 3’ (UTR) sequence of the 14-bp region was discovered to be associated with a lower chance of developing bowel cancer. Meanwhile, sHLA-G levels were greater in the BC group than in the control group. HLA-G polymorphism and expression could therefore play a role in the development of BC, and sHLA-G levels may be used as a diagnostic marker [59]. Furthermore, a study discovered that HLA-E and HLA-G expression are essential factors in determining the prognosis of BC patients. These findings indicate that BC is immunogenic but that it may potentially evade eradication by the host immune system by up- or down-regulating HLA classes Ia and Ib [60].
In the Tunisian population, however, three possible HLA-G 3’UTR polymorphisms
were investigated in BC patients. The +3142 C allele and the +3142 C/C genotype
were shown to be linked to an increased risk of BC. Furthermore, the Del allele
and the Del/Del genotype’s homozygous condition both increase the risk of BC. In
a Tunisian population, however, no statistically significant difference between
HLA-G + 3187 A
A meta-analysis of 14 case-control studies with 2757 cases and 3972 controls found no significant link between HLA-G 14 bp ins/del polymorphism and cancer susceptibility, although HLA-G 14 bp ins/del polymorphism may contribute to BC susceptibility [62]. Additionally, case-control research found no significant allelic or genotypic differences between the case and control groups, while the BC group had a slightly higher allele frequency of the HLA-G 14bp deletion. The HLA-G 14bp InDel polymorphism may function as a potential genetic risk factor for BC development, according to these findings [63].
The results of a meta-analysis of published HLA-G 14-bp association studies
further revealed that other 14-bp associations were inconclusive and that other
sites of variation observed at the HLA-G 3
The degree of evidence supporting the use of serum HLA-G in the diagnosis of BC was recently reviewed in a meta-analysis. This information, however, cannot be utilized to make a diagnosis. This biomarker could be used to assess recurrence and treatment response in the future [65].
In the Tunisian population, the HLA-G +3142 C
The del allele was shown to be significantly associated with the development of BC. The 14-bp ins allele and the ins/ins genotype, on the other hand, were protective against BC. This is the first study to establish a link between the 14-bp HLA-G polymorphism and BC in the Saudi population. The findings are consistent with previous research that found a similar link between HLA-G and cancer in other populations [68]. In addition, patients had more of the 14-bp deletion and the +3010, +3142, and +3187 variations than controls. Two haplotypes of the eight SNPs and deletion/insertion (UTR-1 and UTR-4) are linked to BC when these data are combined. Some HLA-G gene variations in the 3-UTR and associated haplotype combinations have been related to BC [63].
By studying HLA class I and DNA analysis, the expression can be used to predict tumor development and the likelihood of BC recurrence. In 32.5% of BC patients, HLA class I expression was downregulated. HLA class I downregulation was related to lymph node involvement, tumor, nodes, and metastases (TNM) stage, lymphatic invasion, and venous invasion. Patients whose HLA class I was preserved had a longer disease-free interval than those whose HLA class I was deleted [17].
In a study of BC patients and healthy controls, the relationship between KIR genes and HLA alleles was investigated. SSO-PCR was used to type 230 BC patients and 272 healthy controls for HLA class I and KIR genes. The presence of KIR2DL2 inhibitory receptors was higher in BC patients than in healthy controls when the two groups were compared. HLA-C2 and HLA-Bw4 showed no significant differences. However, HLA-C1 was shown to be more common in BC patients. These findings imply that the KIR gene system may have a role in BC [69].
A recent study found that HLA class I-specific immunoglobulin-like receptors (KIRs) control how natural killer (NK) cells get rid of cancer cells. In advanced breast cancer, levels of KIR (aKIR) and HLA-ligand activating combinations 2DS1 + C2 and 3DS1 + Bw4 were higher. All advanced cancer patients who had 2DS1 + C2 or 3DS1 + Bw4 had their Ikir homologous 2DL1 and 3DL1, respectively. In the absence of a putative aKIR homolog, these results show that NK cells that express iKIR to similar HLA ligands play a role in the antitumor response [70].
According to de Kruijf et al. [60], HLA class I and Treg only impact the prognosis in patients treated with chemotherapy and are therefore one of the few indicators of chemotherapy response in early BC patients. Chemotherapy can destroy tumor cells that have retained HLA class I and Treg, allowing cytotoxic T lymphocytes (CTLs) to attack them. As a result, HLA class I and Treg have a strong discriminating ability to predict chemotherapy responses.
PCR-SSP was used to characterize HLA class I genotypic variations in 132 BC patients and 382 healthy controls. The HLA-B7 allele was found to be more common in patients than in controls. These findings join others in finding that HLA-B7 is linked to the development of BC [71]. Furthermore, HLA typing results in 100 Mexican mestizo BC patients and 99 matched healthy controls revealed that there was no significant difference between the two groups in terms of HLA-A. In the case of the group, HLA-B*1501 was shown to be three times more common (Fig. 3). HLA-Cw is neither a risk factor nor a protective factor against the disease [72].
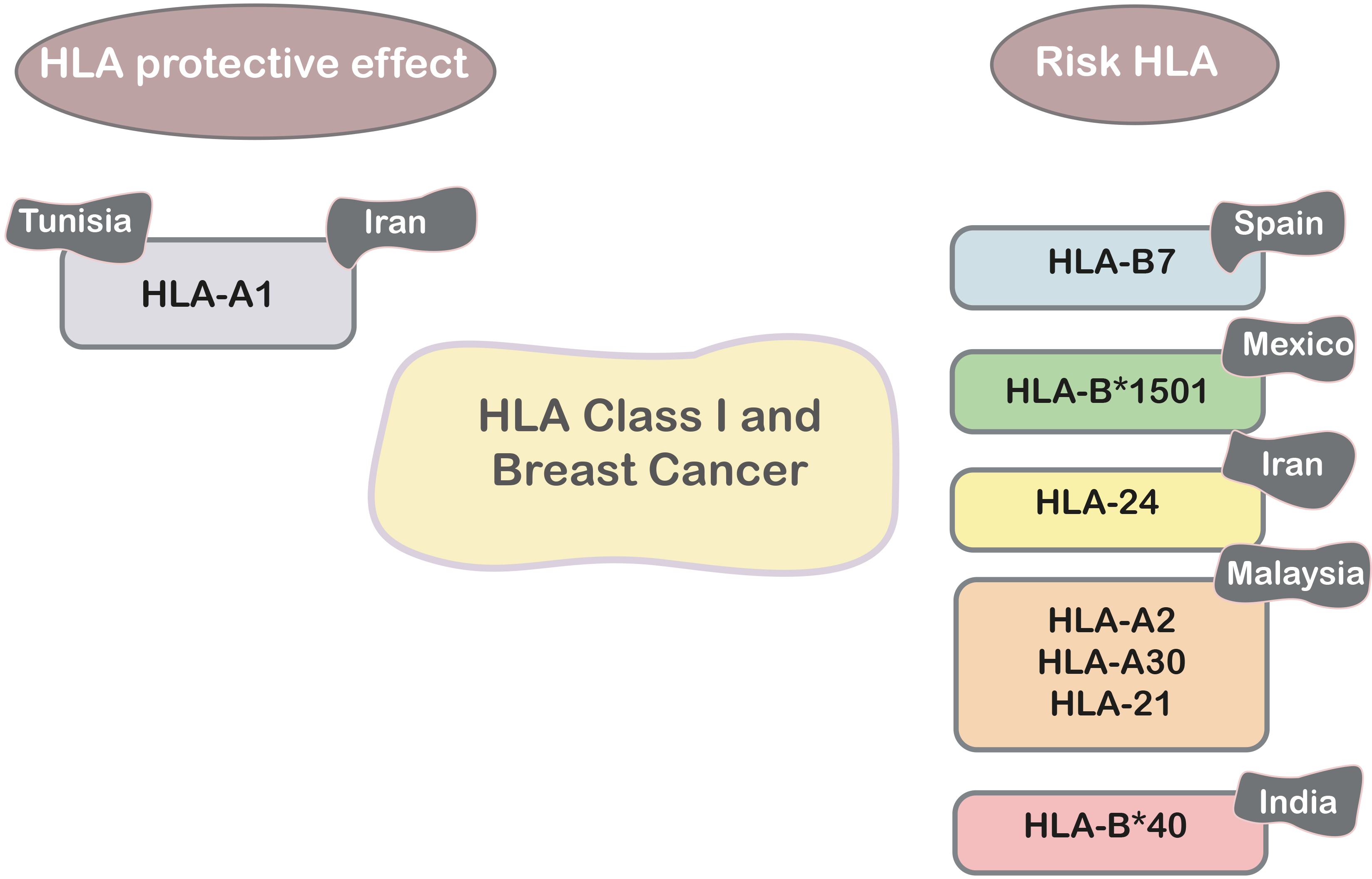
HLA class I and breast cancer in different populations.
Furthermore, there were considerably more patients with the A24 allele than healthy people (38.2% vs. 16.3%). However, in the patient group, HLA-A1 expression was lower than in the control group. The A24 allele appears to be one of the variables that increases an individual’s vulnerability to breast cancer, although further research is needed [73].
59 people with infiltrating ductal breast disease were tested with polymerase chain reaction to find out how often each HLA-A type was found in each site. In patients, HLA-A2/A30 and A2/A31 (5.1%), as well as HLA-A30 (5.1%) and the A31 allele (6.8%), were substantially superior to those of controls (0%). In breast cancer, the HLAA24 allele was associated with lymph node metastasis, whereas the A26 allele was associated with distant metastasis. Finally, HLA-A2, -A30, and -A31 have been linked to BC [74]. In addition, HLA typing revealed a high frequency of HLA-A* alleles 030101-07, 09-11N, 13-16 in BC patients compared to healthy and control patients in 60 subjects: 30 BC patients, 12 patients with benign breast lesions as control patients, and 18 apparently healthy subjects as controls (Fig. 3). The findings suggest that specific HLA-A alleles may play a role in BC etiology [75].
HLA typing was used to identify protective and susceptibility alleles for BC in the Tunisian population. In 64 Tunisian women with BC and 74 unrelated ethnically matched healthy women, Luminex technology was used to perform molecular typing. The results indicated that HLA-A*30 is a risk factor for breast tumor prevalence. However, our findings indicate that HLA A*01 (Fig. 3) confers protection against the most severe form of BC in Tunisia [76].
Further research was conducted among 81 premenopausal BC patients and 160 healthy women from West India who were matched for age and ethnicity. When HLA class I and HLAB*40 alleles were genotyped, patients were shown to have twice as a frequency of HLA-B*40 (16%) as controls. In both research groups, high- resolution typing of HLA-B*40 alleles indicated a preponderance of the HLA-B*4006 allele. Two other notable results are the decreased frequency of HLA-B*08 in patients and the higher proportion of patients with homozygosity at the HLA-Cw gene [77].
According to a recent study, women with the BRCA1 germline mutation are almost
certain to get breast cancer. For 90 young (38 years) and 73 older (
In 176 Caucasian women with early-onset BC and 215 ethnically matched controls, the HLA DPB1, DQB1, DRB1, and DRB3 alleles were genotyped. HLA DQB*03032 was found in 7% of controls but not in any early-onset BC cases. Compared with patients with early breast cancer, HLA DRB1*11 alleles were also overrepresented in controls (16.3%) compared to (3.5%) BC cases. In human breast cancer, the HLA DQB*03032 and HLA DRB1*11 alleles may have a protective effect [18].
In Tunisia, a study examined the relationship between susceptibility to BC and HLA class II indicators. For 70 Tunisian patients, the HLA-DRB1 and HLA -DQB1 loci were molecularly typed. In the Tunisian population, a study of alleles and haplotypes between patients and 70 female control individuals indicates a negative relationship between HLADRB1*07-DQB1*02 and BC incidence [79].
The Turkish population was investigated to see if there was a link between BC and HLA class II alleles. The HLA-DRB1*03, HLADQB1*02, HLA-DRB1*13, and HLA-DQB1*06 alleles are involved in BC prevention and have an excellent prognosis (Fig. 4), whereas the HLA-DRB1*03 and HLADRB1*04 alleles are implicated in a poor prognosis, according to the findings of this study [80].
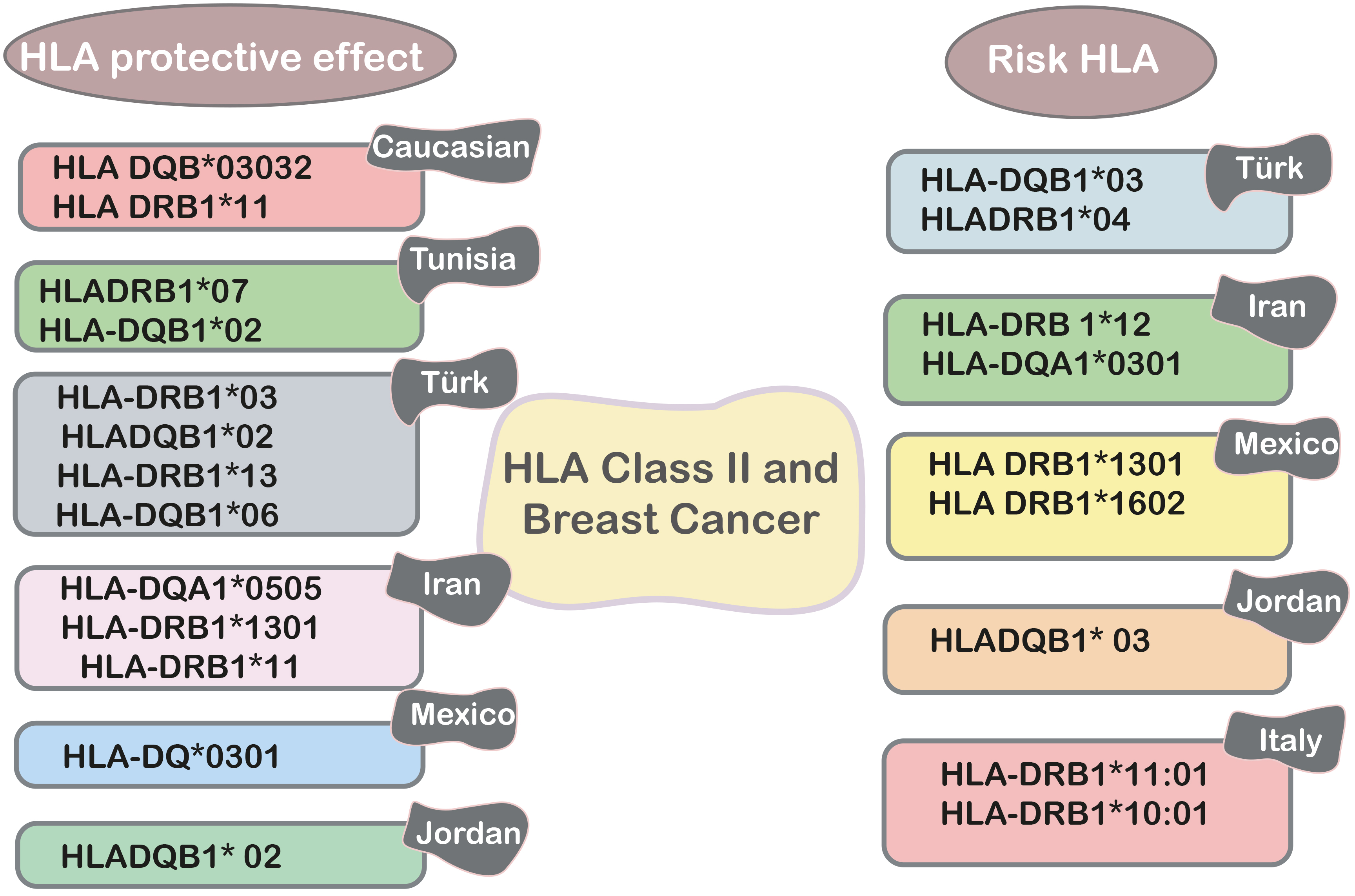
HLA class II and breast cancer in different populations.
PCR-SSP was used to compare the frequency of the HLA-DRB 1 allele in 36 women with operable primary BC from southern Iran to that of 36 women who did not have cancer. The prevalence of the HLA-DRB 1*12 allele was considerably higher in the diseased group than in the control group, according to the findings. Furthermore, the HLA-DRB1*11 allele appeared to be the most common allele in the control group (29.2%) and had a similar distribution in the diseased group (22.2%) [81].
In Iranian women, there is a link between HLA class II alleles and breast cancer. The findings show that the HLA-DQA1*0301 allele is primarily linked to an elevated risk of breast cancer, including the onset of the disease at an early age. HLA-DQA1*0505 and HLA-DRB1*1301 (Fig. 4) are involved in protection [82]. On the other hand, in a case-control study of Mexican mestizo BC patients compared to healthy controls, it was noted that HLA DRB1*1301, which is expressed in seven cases, causes a seven-fold increase in risk, whereas DRB1*1602 has a similar effect. The presence of the DQ*0301 allele, which is considerably more frequent in the control group, may protect against the onset of the disease.
The connection between HLA-DQB1 and HLA-DRB1 MHC class II alleles and disease susceptibility or resistance in Jordanian women was also investigated, with the findings revealing a strong negative association between HLADQB1* 02 alleles and breast malignancies. There was no evidence of a link between HLADQB1* 03, 04, 05, and 06 and HLA-DRB1* 01, 03, 04, 07, 08, 10, 11, 13, 14, and 15. Indeed, HLA-DRB1* 01, 03, 04, 07, 08, 10, 11, 13, 14, and 15 alleles (Fig. 4) offer favorable protection against BC risk in Jordanian women, while HLADQB1* 03, 04, 05, and 06 or HLADQB1* 03, 04, 05, and 06 or HLADQB1* 03, 04, 07, 08, 10, 11, 13, 14, and 15 alleles do not [83].
HLA-DQA1 and -DQB1 polymorphisms were found in BC patients, and the results show that HLA-DQA1 and -DQB1 are not linked to BC in women in southern Taiwan [84]. Additionally, based on current research, a meta-analysis will examine the link between HLA-DRB1 gene polymorphism and breast cancer. This study will look at the early warning signs of a family history of BC and provide medical evidence to explain how the HLA-DRB1 gene polymorphism affects the risk of BC [85].
According to ShKuligina et al. [78] E in BRCA1-directed elderly BCs, the HLA-DBR1*11:03/04 allele was overrepresented (11% vs. 3%). The “young” patient group had substantially higher HLA-DRB1*01:01 and HLA-DQB1*05 allele frequencies. Homozygosity for the HLA II locus limits the repertoire of HLA peptides, which may enhance vulnerability to BC in BRCA1 carriers and present as an early onset of disease [86].
Sequential typing of 156 healthy controls and 47 central Italian BC patients yielded the frequency of HLA-DRB1 alleles. As historical controls, 297 people from the same region were selected. HLA-DRB1*11:01 and HLA-DRB1*10:01 alleles were shown to be substantially related to an elevated risk of breast cancer. HLA-DRB1*11:01 was found to be considerably more common in BC patients than in healthy women and historical controls. These findings show that HLA-DRB1*11:01 and HLA-DRB1*10:01 could be useful indicators for people at risk of BC [83].
However, it has not been shown that carrying the HLA-DRB1*11 or 1*12 alleles increases the risk of BC in patients with BC in Burkina Faso, as shown by Zouré et al. [87]. On the other hand, more research into the HLA alleles that are important in the development of BC could reveal additional insights [87].
The protein known as Retinoblastoma (Rb) is synthesized as a result of the expression of the RB tumor suppressor gene. The prevalence of its expression is extensive in luminal breast cancer. The effectiveness of treatment, including cyclin-dependent kinase (CDK) 4/6 inhibitors, is also crucial [88].
In retinoblastoma, loss of the RB1 gene is thought to have a causal or exacerbating role. Intragenic mutations, methylation-mediated silencing, and chromosomal deletions can all make the RB1 gene inactive. The effects of each are different for different species and cell types. The chance of developing cancer is greatly increased when the RB1 gene is deleted, which may also cause aneuploidy [89].
Corso, G et al. [90] showed that BRCA carriers were found to have an increased risk of developing metaplastic BC compared to all other BC patients who were tested for BRCA genes.
The results of this study emphasize the critical relevance of understanding the complex link between individual HLA polymorphisms and BC risk. These findings have profound implications since they demonstrate the HLA system’s vital involvement in defining the development of breast cancer, potentially through its influence on the immune response directed against malignant cells. However, it is imperative to acknowledge that this study represents only a steppingstone towards unraveling the intricate mechanisms by which HLA polymorphisms modulate BC risk. To truly grasp the full spectrum of the impact of HLA polymorphisms on breast cancer, further comprehensive research is warranted. Future research must delve deeper into the potential use of HLA typing as a strong tool for both accurate BC risk assessment and treatment strategy customization. Innovative techniques to fortify the immune response against tumor cells can be designed by leveraging the knowledge gained from the HLA system, thereby increasing the efficacy of existing treatment strategies. Unraveling the complicated genetic landscape of breast cancer, particularly with respect to HLA polymorphisms, can pave the path for game-changing advances. In summary, this work serves as a significant stimulant for additional scientific investigation, emphasizing the importance of broadening our knowledge and capacities in understanding the genetic roots of breast cancer. This review, by moving science forward, lays the groundwork for a transformative future of improved BC prevention, precise diagnosis, and personalized treatment strategies.
CTLs, Cytotoxic T Lymphocytes; HLA, Human Leukocyte Antigen; HLA-G, Human Leukocyte Antigen-G; HPV, Human Papillomavirus; HRT, Hormone Replacement Treatment; MHC, Human Major Histocompatibility Complex; NK, Natural Killer; PTEN, Phosphatase and Tensin Homolog; RNA, Ribonucleic Acid; SES, Higher Socioeconomic Status; TCR, T Cell Receptor; TNM, Tumor, Nodes, and Metastases; WHO, World Health Organization.
BHG, CIY, YST, and AK, conceptualisation; SA, HAM, HAA, and AB, data collection and analysis; SA, visualisation; AB, AK, MA, and SM, format analysis; SA, AB, AK, MA, and SM, original draft preparation; BHG, CIY, YST, review and editing; BHG, AK, AB, supervision. All authors contributed to editorial changes in the manuscript. All authors read and approved the final manuscript. All authors have participated sufficiently in the work and agreed to be accountable for all aspects of the work.
Not applicable.
Not applicable.
This research was supported by the Deputyship for Research and Innovation, Ministry of Education in Saudi Arabia (project number ISP23-81).
The authors declare no conflict of interest.
Publisher’s Note: IMR Press stays neutral with regard to jurisdictional claims in published maps and institutional affiliations.