- Academic Editor
Background: Toxocara canis (T. canis) is a
helminth parasite of zoonotic and veterinary health significance that causes the
disease known as Toxocariasis. This disease has been associated with conditions
of poverty, especially in tropical climate zones throughout the world. Although
it rarely causes important clinical manifestations, T. canis can lead to
blindness, meningoencephalitis, or other nervous manifestations in humans.
Moreover, some studies show its importance in the development of tumor growth,
which have been associated with the parasite’s ability to modulate the host’s
immune response. While different studies have evaluated the immune response
during this disease, currently, there are no studies where the infection is
analyzed from the perspective of sexual dimorphism. Methods: To evaluate
sex differences in susceptibility, we analyzed lesions and parasite loads in lung
and liver at 7 days post-infection. In addition, immune cell subpopulations were
analyzed in spleen, mesenteric and peripheral lymph nodes. Finally, the
production of cytokines and specific antibodies were determined in the serum.
Statical analyses were performed using a Two-way ANOVA and a post-hoc Bonferroni
multiple comparison test. Results: Female rats had a higher number of
larvae in the liver, while male rats had them in the lungs. The percentages of
immune cells were evaluated, and in most cases, no significant differences were
observed. Regarding the cytokines production, infection can generate a decrease
in Th1 such as IL-1
Sex-associated differences in the immune response can be defined as the variations in cellular and molecular events in the immune system that exist when the organism is exposed to an antigenic challenge caused by a virus, bacteria, or a parasite. These differences have been associated with the role of hormones such as sex steroids that play an important role in the regulation of the immune system [1, 2, 3, 4, 5]. During different studies where the immune response against helminths has been evaluated, previous studies have evaluated the influence of hormones on the immune function. It has been observed that sex (male or female) plays an important role in the regulation of the immune response. Therefore, it has generally been demonstrated that one sex is more susceptible than the other to different parasite infections [6, 7, 8, 9]. In some parasitic infections, hormonal regulation has been associated with larval reactivation that is vertically transmitted (transplacental, lactogenic) from mother to offspring, as is the case of Toxocariasis [10, 11]. However, so far, it has not been described whether there is a dimorphic effect on the immune response that could influence the migration of the larvae towards organs such as the uterus and the mammary gland, thereby modifying susceptibility in males and females.
Toxocara species are parasitic nematodes whose definitive hosts are dogs and cats. Toxocara canis (T. canis) infects dogs as the definitive host, whereas Toxocara cati (T. cati) is found in cats. Additionally, this parasite can affect humans; therefore, it is considered a zoonotic disease; as well as other mammals, birds, and invertebrates among others, causing the disease known as Toxocariasis [11, 12, 13]. In humans, the infection can go from asymptomatic to produce different clinical manifestations caused by the migration of T. canis larvae through the bloodstream and other organs. This disease can be classified as Visceral Larva Migrans (VLM), Neurotoxocariasis (NT), Ocular Larva Migrans (OLM), and Covert Toxocariasis (CT) [14, 15, 16].
During T. canis infection, the predominant immune response is a Th2 type, although a dichotomy has also been mentioned in terms of the polarization of the immune response. It is possible to find molecules of both the Th1 and/or Th2 type. This response may be influenced by aspects such as the host species (dog, cat, mouse, rat, human), time of infection (acute or chronic), and target organ (lung, liver, brain), among others. However, it is worth mentioning that, currently, the immune response during T. canis infection has not been analyzed from a sex perspective. In general, the immune response is assembled against somatic antigens and excretory-secretory antigens (TcES-Ag) [17, 18, 19]. This response is characterized by a strong adaptive immune response, where a Th2-type response predominates during the chronic infection. The Th2 response is characterized by the production of cytokines such as interleukin (IL) IL-4, IL-5, IL-6, IL-13, IL-33, and regulatory cytokines such as IL-10, among others [17, 18, 19, 20, 21]. However, during the acute phase of infection, a strong pro-inflammatory response takes part in the immunological profile, where a mixed response with inflammatory cytokines of innate origin, together with Th17 and Th2 type cytokines has been reported [22].
Th2 cytokines are involved in the activation of other immune cell subpopulations such as mast cells, macrophages, eosinophils, and the secretion of Immunoglobulins (Ig) IgE and IgG1 [19, 20]. These cytokines and Ig are associated with leukocytosis, peripheral blood eosinophilia, eosinophilic infiltration around larval sites of migration, as well as specific antibody production [22].
Macrophages also play an important role during infection, and macrophages
obtained from mice within ten days of infection with T. canis, and
cultured in vitro with lipopolysaccharide, have been reported to produce
higher amounts of IL-10 and tumor growth factor-beta (TGF-
In addition, different studies have shown that the immune system can be regulated by different hormones, such as sex steroids [30, 31, 32, 33, 34]. On this basis, differences in susceptibility and the associated immune response have also been reported in some other parasitic diseases [6, 8, 9, 35, 36, 37, 38, 39]. Nonetheless, as far as we are concerned, there is a lack of information that demonstrates the immune modulation caused by sex steroids during Toxocariasis. Within this framework, this study aims to evaluate susceptibility, immune response, and immune effector mechanisms during acute T. canis infection from the point of view of immune sexual dimorphism, to describe an immunomodulatory effect that could increase susceptibility to infection to this parasite in male or females.
The protocol for the use and care of the animals was endorsed by both Institute’s Animal Care and Use Committee (Comité Institucional para el Cuidado y Uso de Animales de Laboratorio, CICUAL, permit number 201-2016) following the official Mexican regulations (NOM-062-ZOO-1999). These regulations are in accordance with the recommendations outlined in the Guide for the Care and Use of Laboratory Animals of the National Institute of Health of the USA, ensuring compliance with established international regulations and guidelines.
Twenty-eight male and Twenty-eight female Wistar rats of sixty days old, were
obtained from the experimental animal facility of the Instituto de
Investigaciones Biomédicas, Universidad Nacional Autónoma de México.
The rats were divided into four groups and allocated in polycarbonate boxes (50
cm L
T. canis eggs were obtained from adult parasites donated by Centro de
Control Canino de Cuautitlán, Estado de México. Female worms were
separated, rinsed in tap water, and placed in phosphate buffer solution (PBS)
with a pH around 7.2–7.4. Subsequently, uteri were collected through an incision
in the first third of the body and placed in a physiological saline solution.
Eggs were obtained using a fine pore filter. The ova were washed several times in
PBS and centrifuged at 3250
Male and female rats were infected with 1000 T. canis larvated eggs at sixty days old. The inoculum for the infection was washed three times to eliminate the PBS/formaldehyde solution. Eggs were then resuspended in PBS and concentrated to 1000 larvated eggs per ml. The infection was performed by intragastric administration using a type of Foley metallic probe. Seven days later, the rats were humanely euthanized using sevoflurane (Sevorane®) anesthetic overdose.
Right after the rats were euthanized, blood was collected in Serum Separation
Tube (SST)-Vacutainer tubes BD (BD
Biosciences, San Diego, CA, USA). After collection, the blood was allowed to clot
by leaving it undisturbed at room temperature for 3 minutes and the clot was
removed by centrifuging at 2000
The lungs and liver of infected rats were weighed, and small pieces of these
organs were obtained by cutting them off. The tissue was then macerated in PBS
1
For the assay, spleen, mesenteric lymph nodes (MLN), inguinal, and axillar
peripheral lymph nodes (PLN) were collected from rats immediately after
euthanasia. The samples were disaggregated using a sterile nylon mesh (50
µm) and a syringe plunge in PBS at 4 °C. The splenic cell
suspension was centrifuged at 182
For extracellular staining, cells were incubated with a primary antibody
solution for 10 min at 4 °C. The following selected primary antibodies
were used: AF 488 anti-rat CD3 (Biolegend, Clone 1F4, San Diego, CA, USA) (1:100), PE/Cy5 Mouse
anti-rat CD4 (BD bioscience. Clone Ox-35) (1:300), PE Mouse anti-rat
CD8
Eight different cytokines: IL-1
TcES-Ag was obtained by culturing the larvae. T. canis embryonated eggs
were centrifuged at 3250
The integrity and purity of antigens were determined by SDS-PAGE and Coomassie staining. Protein content was quantified using the Bradford method. Different TcES-Ag dilutions were performed in PBS and mixed with the Bradford reagent (Bio-Rad, Hercules,CA, USA). The protein concentration was determined by comparing the optical density (O.D.) of the sample to a standard curve (10, 20, 40, 60, 80, and 100 µg/mL) made with bovine serum albumin (BSA, SIGMA-ALDRICH, St Louis, MO, USA). The concentration of total antigens obtained in the test was 235.14 µg/mL.
To determinate specific antibodies, 96 wells flat-bottom polystyrene plates (Maxisorp, NUNC Labs, Norristown, PA, USA) were sensitized with 1 µg/mL TcES-Ag in bicarbonate buffer (pH 9.6) for 24 hours at 4 °C. Subsequently, the plates were washed three times with 0.01% PBS-Tween 20 (SIGMA-ALDRICH), blocked with 3% bovine serum albumin (BSA), and stored at 4 °C for 24 hours. After the blocking time finished, the plates were washed three times with PBS-Tween 20 at 0.01% and stored at 4 °C. For each sample, 50 µL of serum/well was used in duplicate, diluted 1:200 with PBS, and incubated at 37 °C for 2 hours. Subsequently, the plates were washed three times with PBS-Tween 20 at 0.01%, and 50 µL/well of secondary antibody [1:1000 peroxidase-conjugated AffiniPure Goat Anti-Rat IgG (H+L), Jackson Immunoresearch, West Grove, PA, USA] was added and incubated at 37 °C for one hour. The plates were washed five times with the same solution (PBS-Tween 20 at 0.01%), and color development was achieved using 0.05% o-phenylenediamine (OPD) and 0.001% hydrogen peroxide in citrate buffer (SIGMA-ALDRICH). The plates were incubated for 15 min in total darkness at 37 °C, and then, 50 µL/well of 0.06% orthophosphoric acid was added. Plates were read at 492 nm with 15 seconds of agitation in an ELISA reader (Stat Facs 4200, Awareness Technology, Palm City, FL, USA).
Data are presented as mean
The number of lesions and larvae number was significantly different depending on
sex. In males, lesions and larvae counts were higher in the lungs seven days
post-infection. In contrast, in females, the extent of the lesions and the number
of recovered larvae were higher in the liver (Fig. 1a,b). Combining the
mean number of larvae obtained in both organs we observed statistically
significant differences (p
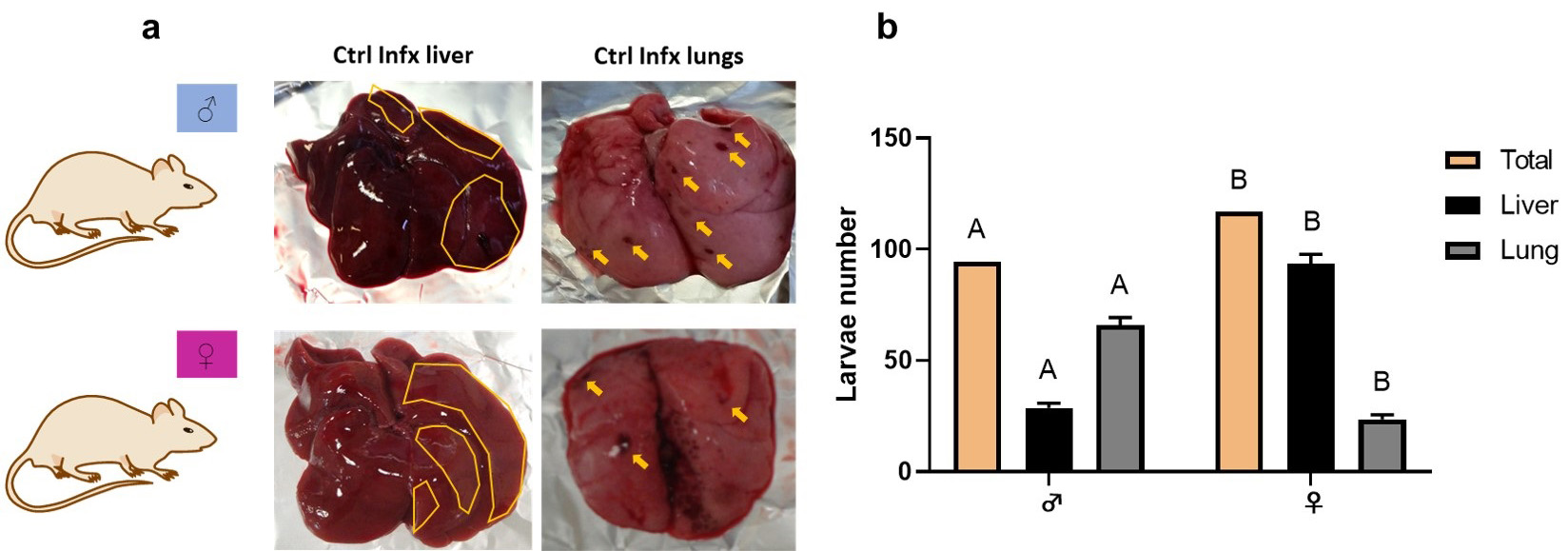
Macroscopic lesions and larvae number of T. canis in
the lungs and liver. (a) Liver and lungs injuries. In the liver, the areas of
damage are outlined in yellow. In the lungs, yellow arrows point to areas of
damage. (b) Total larval number in both organs and larval number in liver and
lungs. Bars represent the mean
Because sex steroids can modulate the immune response in infections, we decided
to evaluate the immune response during a T. canis infection as an
antigenic challenge. Fig. 2 shows Macrophages (M
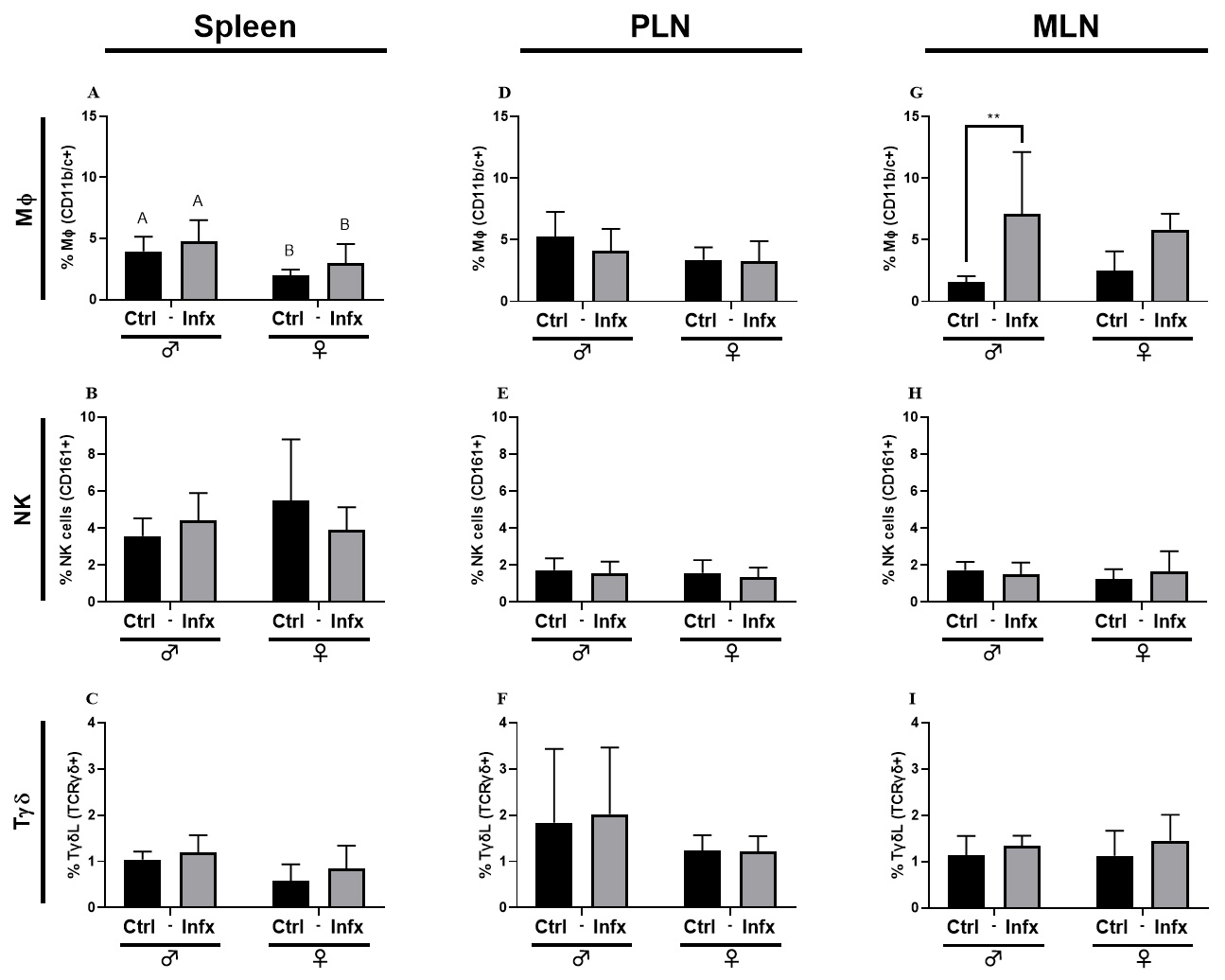
Percentage of the innate immune cells subpopulations in male and
female rats control or infected with T. canis. (A) M
Fig. 3 shows the percentage of total T cells, T helper lymphocytes
(ThL) and T cytotoxic lymphocytes (TcL) in the spleen, PLN and MLN. In the
spleen, the infection generated an increase in the percentage of ThL only in
males (p
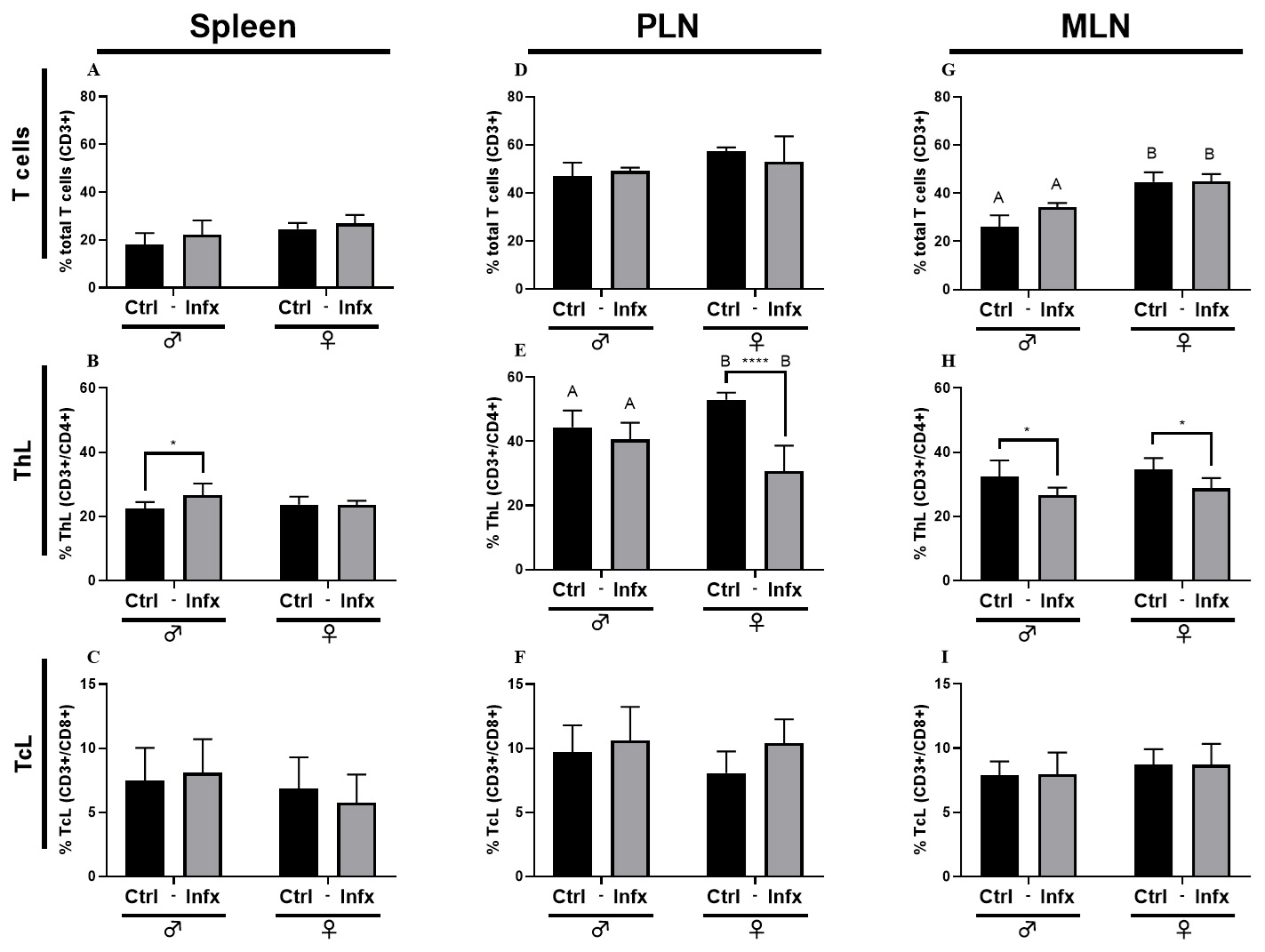
Percentage of T lymphocytes in male and female rats control or
infected with T. canis. (A) Total T cells (CD3
Finally, in B lymphocytes (BL), the infection generates an increase in the
number of these cells (p

Percentage of B Lymphocytes (BL) in male and female rats control
or infected with T. canis. (A) BL (CD45RA+) in the spleen. (B) BL
(CD45RA+) in PLN. (C) BL (CD45RA+) in MLN. Bars represent the mean
To evaluate cell response in the immune system, we analyzed the production of
cytokines during T. canis infection in both sexes. For Th1 cytokines,
the infection reduces the concentration of IL-1
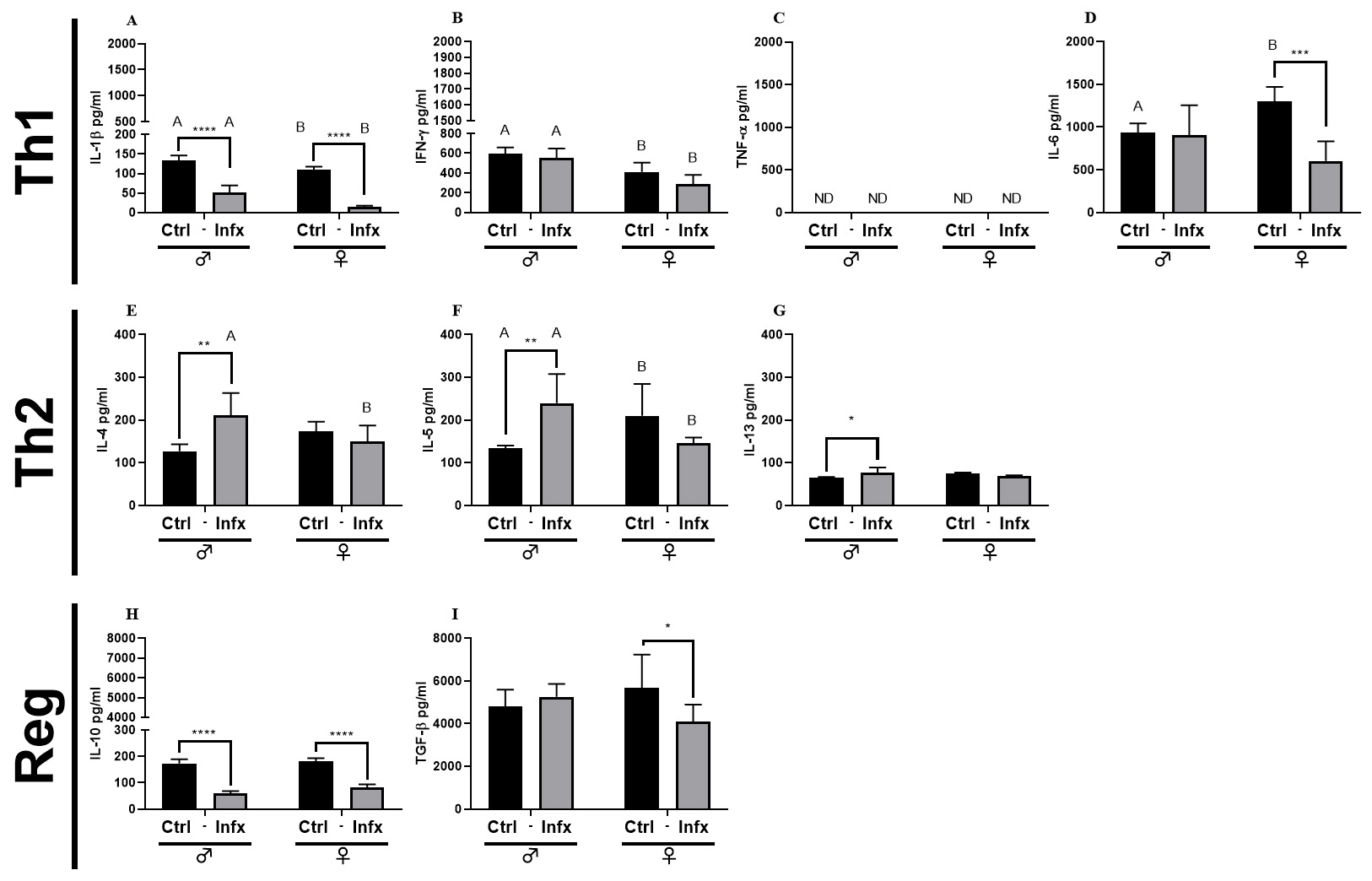
Analysis of systemic soluble factor expression in serum
of male and female rats, control, or infected with T. canis.
(A) IL-1
Since the production of specific antibodies is an important strategy for the
control of helminths, we decided to determine the presence of these antibodies in
the serum of the animals. The infection caused an increase in the production of
specific Ig-G anti-T canis in both sexes (p
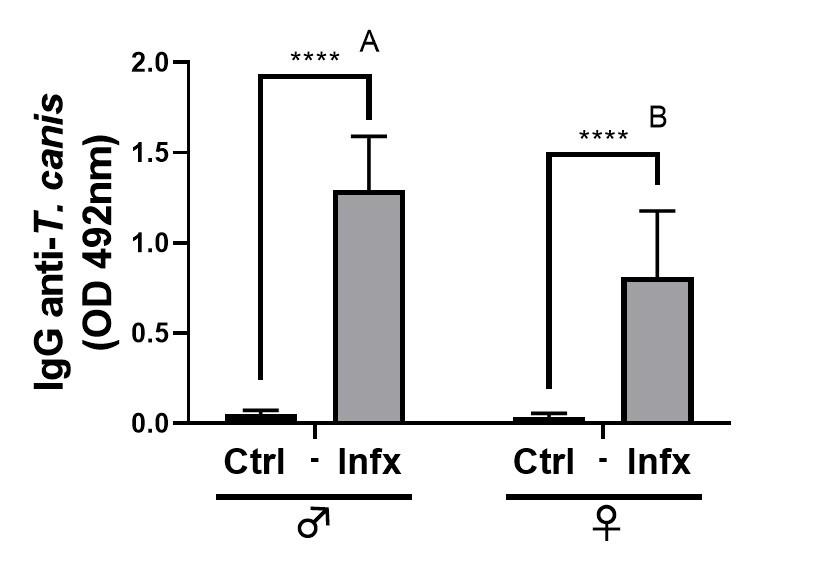
Analysis of specific anti-T. canis antibody
production in control and infected male and females. Bars represent the mean
In this study, we estimated the effect of sex on susceptibility to T. canis infection by evaluating the immune response during the acute phase of the infection in a rat model. The initial assessment of susceptibility focused on the analyzing parasitic loads in organs such as lungs and liver. We chose to evaluate these organs because, during the time of infection, migrating somatic larvae are present throughout them [13, 14, 15, 16, 17, 18, 19, 20, 21, 22, 23, 24, 25, 26, 27, 28, 29, 30, 31, 32, 33, 34, 35, 36, 37, 38, 39, 40] (Fig. 1B). Our data align with findings reported by Lescano et al. [40], who infected male Wistar rats with 500 larvated eggs, observing that the number of larvae recovered at eight days post-infection in the liver and lung was 12.3 (2.46% of total larvated eggs) and 35.7 (7.14% of total larvated eggs), respectively. In studies with other species of Toxocara (T. cati), by Santos et al. [41], (2009) infected adult male Wistar rats with 300 larval eggs, reporting a recovery of 4 larvae (1.33% of total larvated eggs) from the liver and 13.6 larvae (4.53% of total larval eggs) from the lung. Additional experiments by dos Santos et al. [42], (2017) evaluated the pattern of somatic larvae migration in male and female Wistar rats infected with 300 larvated eggs. They found that seven days post-infection, the recovery of larvae in males was 13 in the liver (4.33% of total larvated eggs) and 2 in the lungs (0.66% of total larvated eggs). In females, the number of larvae recovered from liver was 27.5 (9.16% of total larvated eggs) and 1.5 in the lungs (0.5% of total larvated eggs). The increase in the number of larvae at the hepatic level in females was maintained from seven to 60 days post-infection. Moreover, the total number of larvae recovered in these organs was higher in females than in males, indicating that females are more susceptible than males to T. canis infection [42]. These results align with those reported by our research group, where we observed a dimorphic distribution of larvae in the liver and lungs, with females having a higher number of larvae in the liver than males. Although these differences in parasitic loads at the pulmonary level shows statistically significant differences, with males having a greater number of larvae than females. Additionally, the total number of larvae in both organs was higher in females than in males (Fig. 1B). Even though it remains unclear why these differences occur in certain larvae located in these organs, it has been previously reported that differences in enterohepatic circulation exists in rats between both sexes. For instance, a higher recirculation and consequently, a reduced excretion of some molecules have been demonstrated [43]. This fact could allow the larvae to remain in the liver of females for a longer period compared to males. The present results differ from the prevalence reported in definitive hosts, where it is mentioned that males are more susceptible to infection than females. This difference may be attributed to the fact that in males, transmission is completed only through intestinal patent infection, whereas in females, a larval reactivation phenomenon occurs with consequent transplacental and lactogenic transmission [44]. On the other hand, additional factors, such as the immunosuppressive effect of testosterone, may contribute to these differences [45]. However, it is worth highlighting that in these reports, the age of the animals is not mentioned or no statistically significant differences were found between both sexes. Additionally, in this study, paratenic hosts, namely rats, were used, and the parasite does not develop into its adult form in this rodent host; it can only be transmitted to the offspring by the lactogenic way [46] or by ingestion of prey infected with somatic larvae.
Since the immune response plays a key role in the development of resistance or
susceptibility to various diseases, whether of non-infectious or infectious
origin, we decided to evaluate the primary cells of the innate and adaptive
immune response responsible for controlling helminth infections in the spleen,
MLN, and PLN. Concerning cells from the innate response, only M
As mentioned earlier, the Th2 response plays a crucial role in orchestrating the
immune response during helminthic infections. Here, we report a decrease of Th1
cytokines, IL-1
Finally, sexual dimorphism involves the biological differences between males and females of the same species, including developmental, physiological, and pathological dissimilarities as presented in here. The scientific significance of the present piece of research, it is of high priority to people interesting in an update on sex differences in parasitic infections, and biological factors of risk, and explains the latest sex-associated factors for T cannis Toxocariasis first, and ultimately for many helminth infections that are dimorphic in their presentations.
Our study demonstrates that T. canis infection is dimorphic and affects females more than males. This is attributed to a polarization of the inadequate immune response, which is reflected as a higher parasite load in this sex.
The datasets generated and analyzed during the current study are included in the present manuscript. Furthermore, they are available from the corresponding author on request.
VHDRA, KENC and JMM conceptualization. VHDRA, YAC and CAGC methodology and validation. VHDRA, YAC, CAGC and JMM formal analysis. VHDRA, YAC and JMM investigation and resources. VHDRA, YAC, CAGC, KENC, and JMM data curation. VHDRA writing—original draft preparation. VHDRA, YAC, CAGC, KENC, and JMM writing—review and editing. VHDRA and JMM supervision. JMM project administration. VHDRA, YAC, KENC, and JMM funding adquisition. All authors contributed to editorial changes in the manuscript. All authors read and approved the final manuscript. All authors have participated sufficiently in the work and agreed to be accountable for all aspects of the work.
The protocol for the use and care of the animals was endorsed by both Institute’s Animal Care and Use Committee, (Comité Institucional para el Cuidado y Uso de Animales de Laboratorio (CICUAL), permit number 201-2016) following the official Mexican regulations (NOM-062-ZOO-1999).
Not applicable.
This research was funded by Grants from Programa de Apoyo a Proyectos de Investigación e Innovación Tecnológica, (PAPIIT), Dirección General de Asuntos del Personal Académico, (DGAPA), Universidad Nacional Autónoma de México, (UNAM) to Víctor Hugo Del Río-Araiza Grant IA-207023. Grant IN-202723 to Jorge Morales-Montor and Grant IN-218720 to Yazmín Alcalá-Canto.
The authors declare no conflict of interest. Given his role as Guest Editor, Jorge Morales-Montor had no involvement in the peer-review of this article and has no access to information regarding its peer review. Full responsibility for the editorial process for this article was delegated to Giuseppe Murdaca.
Publisher’s Note: IMR Press stays neutral with regard to jurisdictional claims in published maps and institutional affiliations.