- Academic Editor
†These authors contributed equally.
Background: Gastric cancer (GC) stands as one of the most prevalent cancer types worldwide, holding the position of the second leading cause of cancer-related deaths. Gastric lesions represent pathological alterations to the gastric mucosa, with an elevated propensity to advance to gastric cancer. Limited research has explored the potential of stem cells in the treatment of gastric lesions. Methods: This study aimed to explore the potential of intravenous transplantation of labeled bone marrow-derived mesenchymal stem cells (BMMSCs) to inhibit the progression of precancerous gastric lesions. Results: In the gastric lesion disease model group, the rat tissue exhibited noteworthy mucosal atrophy, intestinal metaplasia, dysplasia, and inflammatory cell infiltration. Following the infusion of BMMSCs, a notable decrease in gastric lesions was found, with atrophic gastritis being the sole remaining lesion, which was confirmed by morphological and histological examinations. BMMSCs that were colonized at gastric lesions could differentiate into epithelial and stromal cells, as determined by the expression of pan-keratin or vimentin. The expression of vascular endothelial growth factor was significantly elevated following BMMSC transplantation. BMMSCs could also upregulate the production of humoral immune response cytokines, including interleukin (IL)-4 and IL-10, and downregulate the production of IL-17 and interferon-gamma, which could be highly associated with the cellular immune response and inflammation severity of the lesions. Conclusions: BMMSC transplantation significantly reduced inflammation and reversed gastric lesion progression.
Precancerous gastric lesions, hereafter referred to as gastric lesions, are identifiable pathological changes to the gastric mucosa that are more likely to develop into gastric cancer (GC) [1]. Intestinal metaplasia and dysplasia are two common types of gastric lesions caused by long-term injury and repair cycles. Gastric carcinogenesis can be described as a series of sequential phases, and it is widely accepted that GC occurs following the mucosal atrophy-intestinal metaplasia-dysplasia disease course. Globally, GC is one of the most common malignancies and is also the fourth leading cause of cancer-related deaths [2].
Emerging strategies in stem cell transplantation hold promise as an effective approach to alleviate patient suffering and enhance the treatment of a diverse range of diseases and injuries. Mesenchymal stem cells (MSCs) represent a particularly promising tool for innovative clinical concepts supporting cellular therapy. Initially identified in bone marrow (BM), MSCs demonstrate robust self-renewal and multilineage differentiation potential [3]. Furthermore, they express various surface markers typical of stromal cells, endothelial cells, and epidermal cells [4]. MSCs exhibit the ability to migrate towards ischemic or injured tissues and organs through the circulatory system [5]. They can also be recruited directly to gastrointestinal tissue, participating in both physiological regeneration and pathophysiological repair [6].
Numerous cancer types arise from chronic inflammation, as it has the potential to alter the local microenvironment, thereby increasing the risk of cancer [7, 8]. However, a microenvironment conducive to tissue repair serves as the foundation for disease recovery. Typically, MSCs can regulate immune responses, trigger the production of growth factors and secretion of cytokines to inhibit an inflammatory response [9], improve the microenvironment [10], and control the differentiation of stem cells [11]. However, the biological effects of colonized MSCs may be weakened and their proper differentiation may be compromised when the microenvironment changes during sustained inflammation [12]. MSC transfusion has been exhibited to reverse inflammation-related gastric lesions by regulating and improving the microenvironment [13].
Previous studies have explored predictive factors for prolonged remission, and in young patients with type 1 diabetes mellitus, residual beta cell function predicts clinical response after autologous hematopoietic stem cell transplantation [14, 15]. A previous clinical study on the treatment of ulcerative colitis (UC) using autologous bone marrow whole stem cell transplantation has exhibited to be a safe and effective alternative treatment [16]. As there is strong evidence that bone marrow-derived mesenchymal stem cells (BMMSCs) contribute to the repair of various gastrointestinal injuries [17], the present study aimed to examine their ability to repair gastric lesions. By injecting BMMSCs into the tail vein of rats with a gastric lesion model, the colonization and differentiation of BMMSCs at sites of local gastric lesions were confirmed. The effects of BMMSCs on inflammation-associated cytokines were also investigated, and BMMSCs could serve as optimal candidates for stem cell therapy aimed at impeding the advancement of gastric lesions, leveraging anti-inflammatory and immunomodulatory mechanisms. This study may provide preliminary data and establish the foundation for BMMSC-based therapy in the context of gastric precancerous lesions.
BM aspirates were obtained from the tibia and femur of male Wistar rats using
21-gauge needles containing Dulbecco’s modified Eagle’s medium (DMEM)
supplemented with 1% antibiotics and antimycotics. After filtering through a
nylon mesh (50 µm) and washing with DMEM, the cells were centrifuged,
re-suspended, and plated in a petri dish (100 mm) in DMEM containing 1%
heat-inactivated fetal calf serum. Cells were incubated at 37 °C with 5% CO
The appropriate concentration of CellTracker™ CM-Dil dye (1
µg/mL; C7000; Invitrogen Corp., Carlsbad, CA, USA) was established as
suitable through preliminary experiments. Cells from the third passage were
harvested and counted, and CM-Dil (3 µL) working solution was utilized to
stain 1
Wistar rats of the male gender, aged 6 weeks (160–180 g) were provided by the Laboratory Animal Center of the Fifth Medical Center, Chinese PLA General Hospital (Certification number SCXK-JUN 2007-004). The room temperature was set at 20
Six-week-old male Wistar rats were randomly divided into two groups: control
group (n = 4) and model group (n = 22). Rats in the control group were fed
standard rat chow and tap water ad libitum. In addition to standard rat
chow, rats in the model group also had access to a fresh solution of MMNG
(N-methyl-N
Rats in the control and nontransplantation groups were killed one week after the last stem cell transplantation, and blood was collected from the heart chambers after intraperitoneal anesthesia with 10% chloral hydrate (0.3 mL/100 g). After the blood was centrifuged for 10 min at 3000 rpm, serum was collected and stored at –20 °C. In addition to blood, the entire glandular stomach was resected and incised rapidly along the greater curvature. For background staining and immunohistochemistry, the gastric tissue was fixed with 4% paraformaldehyde before paraffin-embedding and sequential slices were obtained. Lesions, inflammatory cell infiltration, atrophy, intestinal metaplasia, and dysplasia were assessed based on histological criteria from hematoxylin and eosin-stained gastric mucosa samples. The pathological changes were graded as mild, moderate, or severe based on a pathological grading system [18]. In the transplantation group, rats were anesthetized and killed for macroscopic and microscopic analyses after the last injection of labeled cells. Samples of the glandular stomach that were left after the previously described tissue collection were embedded in Tissue-Tek OCT compound (4583, Sakura Co., Ltd., Tokyo, Japan) and frozen in liquid nitrogen for subsequent cryostat sectioning and immunofluorescence staining.
Continuous frozen sections (7 µm) were permeabilized with Triton X-100
(1%) in PBS (pH 7.4) for 20 min, followed by incubation in blocking buffer
containing goat serum (10%) for 30 min. For immunofluorescence staining, the
sections were subsequently treated with anti-vimentin antibody (SC-6260,
monoclonal mouse, 1:200 dilution, Santa Cruz Biotechnology) or pan-keratin
antibody (4545S, monoclonal mouse, 1:300 dilution, Cell Signaling Technology,
Danvers, MA, USA) overnight at 4 °C. Fluorescein
isothiocyanate-conjugated goat anti-mouse IgG antibody (SC-2010, Santa Cruz
Biotechnology, Dallas, TX, USA) was utilized as the secondary antibody (1:100;
Jackson ImmunoResearch Laboratories, West Grove, PA, USA) and sections were
incubated for 1 h at 37 °C. Nuclei were visualized using
4
Immunohistochemistry was performed to detect the expression level of vascular
endothelial growth factor (VEGF). Briefly, paraffin-embedded sections were
deparaffinized and rehydrated. After blocking endogenous peroxidase activity with
H
The presence of interleukin (IL)-17 (BMS8635FF; eBioscience, San Diego, CA,
USA), IL-4 (51-9004113; BD Biosciences, Franklin Lakes, NJ, USA), IL-10
(51-9004112, BD Biosciences), and interferon-gamma (IFN-
Two pathologists specializing in pathology for more than 5 years assessed gastric tissue lesions under light microscopy. The diagnostic criteria for histological changes in the gastric mucosa refer to the classification and diagnostic criteria of experimental gastric precancerous lesions. The pathological indicators were summarized as follows, encompassing the assessment of gastric mucosal injury, inflammation, atrophy, intestinal metaplasia, and atypical hyperplasia.
Gastric mucosal injury: The degree of gastric mucosal injury was categorized into four levels.
Mild: Superficial injury of gastric mucosal surface epithelial cells, scored 1 point; Moderate: Damage above the gastric pit, manifested as superficial erosion, scored 2 points; Severe: Damage below the gastric pit, manifested as deep erosion, scored 3 points; Ultra Severe: Full-thickness necrosis and desquamation of gastric mucosa, manifested as acute ulcer, scored 4 points.
Gastric mucositis was semi-quantitatively assessed. Five visual fields were observed for each gastric body and gastric antrum slice under low magnification. Based on the degree of inflammatory cell infiltration, it was classified into the following levels.
Grade 0: No inflammation, scored 0 points; Grade 1: Multiple chronic inflammatory cell infiltrates in the gastric mucosal epithelium or at the base of the proper gland, scored 1 point; Grade 2: More inflammatory cells infiltrating from the gastric mucosal epithelium to the mucosal muscularis, scored 2 points; Grade 3: Piles of inflammatory cell aggregates in the gastric mucosa, scored 3 points.
Wistar rats of the male gender, aged 6 weeks (160–180 g), and meeting the criteria of Specific Pathogen-Free (SPF) status were utilized in the study. These rats were obtained from and maintained by the Animal Laboratory Center of the Fifth Medical Center, Chinese PLA General Hospital, under license number SCXK (Military) 2007-004. All experimental procedures were conducted with the approval of the Ethics Committee of the Animal Facility of the Chinese PLA General Hospital and adhered to the established guidelines for the care of laboratory animals [19].
Five different fields of view were randomly selected from each
immunohistochemical section under a high-power microscope (400
All the data were expressed as the mean
In this study, labeled MSCs were injected into the tail vein of rats to assess healing in a model of gastric lesions. Pathological changes of the gastric mucosa were observed in the transplantation group (transplant) and compared to the non-transplantation group (model) and the untreated normal group (control). Both morphological and pathological changes were investigated. The percentages of gastric mucosal pathological changes observed in the three groups of rats are presented in Table 1.
Control group | Model group | Transplant group | |
Normal | 50% (2/4) | 0% (0/11) | 0% (0/11) |
Inflammation | 50% (2/4) | 100% (11/11) | 100% (11/11) |
Atrophic gastritis | 0% (0/4) | 64% (7/11) | 45% (5/11) |
Intestinal metaplasia | 0% (0/4) | 27% (3/11) | 0% (0/11) |
Dysplasia | 0% (0/4) | 73% (8/11) | 0% (0/11) |
In the model group, atrophic gastritis, intestinal metaplasia, and dysplasia were all observed along with inflammatory cell infiltration. In the transplant group, a significant reduction in gastric lesions was found, with atrophic gastritis being the sole identified lesion. This finding was confirmed through both morphological and histological assessments (Fig. 1).
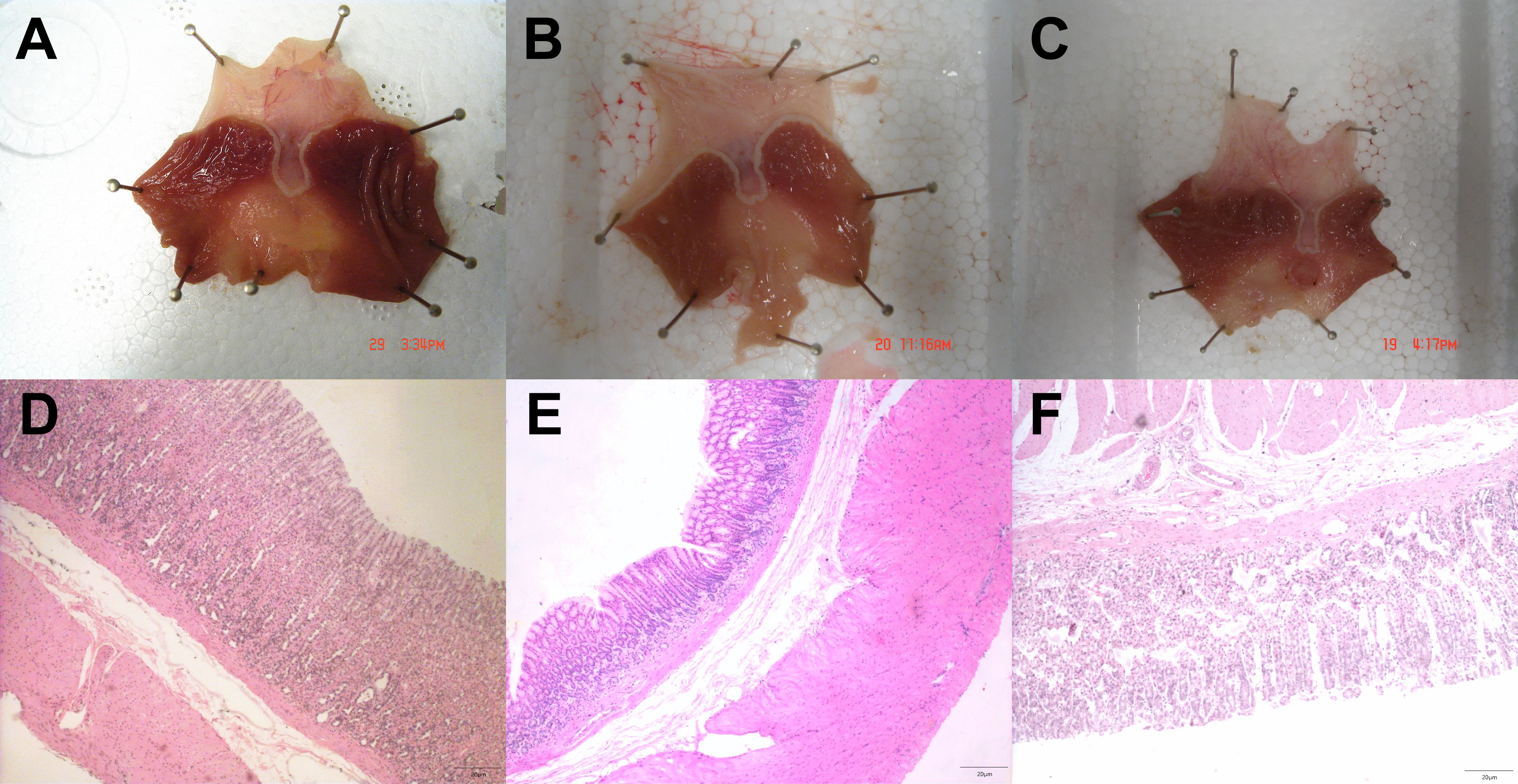
Gastric precancerous lesions were less severe in mice treated with bone marrow-derived mesenchymal stem cells (BMMSCs) that were administered through the tail vein of rats. (A–C) representative images from morphological observations of the rat gastric mucosa in normal (A), gastric lesion model (B) and BMMSC transplant (C) groups. (D–F) Hematoxylin and eosin-stained images from frozen pathological section of rat gastric mucosa in normal (D), gastric lesion model (E), and BMMSC transplant (F) groups.
Macroscopically, the normal rat gastric mucosa exhibited good elasticity, a smooth surface, and regular, light-red folds (Fig. 1A). In contrast, the gastric mucosa in rats of the gastric lesion disease model appeared pale with diminished folds (Fig. 1B). The gastric mucosa in the transplant group showed improved morphological changes compared with the model group, and signs of mucosal repair, such as increased mucosal folds were observed (Fig. 1C). Microscopically, the normal rat mucosa exhibited integrated epithelium with regular cell size, well-organized gland cells, and a small number of scattered lymphocytes (Fig. 1D). The gastric lesion model group exhibited clear indications of mucosal atrophy, intestinal metaplasia, and dysplasia (Fig. 1E). Exfoliation of epithelial cells, reduced glands in the lamina propria, and glandular structure damage with noticeable inflammatory cell infiltration were characteristic pathological changes in this group. However, intestinal metaplasia and dysplasia were not found in the transplant group (Fig. 1F). Further investigation also revealed that MSC transplantation reduced the severity of gastric mucosal inflammation (Fig. 2). An intensified inflammatory response was detected in both the antrum and body of the gastric mucosa (Fig. 2).
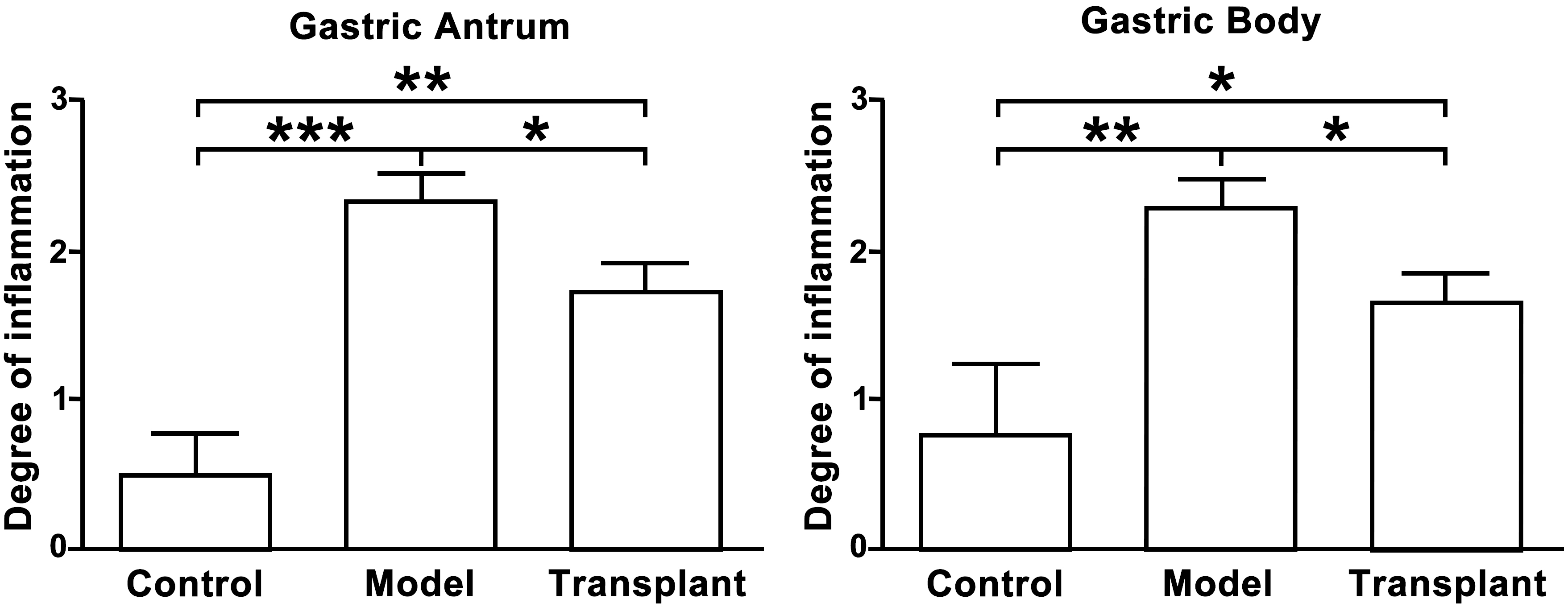
Inflammation was reduced in the rat stomach during a gastric
lesion model after the injection of bone marrow-derived mesenchymal stem cells
(BMMSCs). The severity of inflammation within the gastric antrum (left)
and gastric body (right) of the control, model, and BMMSC transplant groups.
*p
Representative images from frozen gastric tissues following MSC transplantation are illustrated in Fig. 3. Cells with red fluorescence (CM-Dil-labeled cells) were MSCs and were observed at both the gastric body and antrum, while no cells with red fluorescence were found at the gastric fundus. Experiments on control rats were performed in parallel and no cells with red fluorescence were identified in any gastric tissues (Fig. 3). These results demonstrated that MSCs could colonize gastric lesions in rats at the gastric body and antrum.
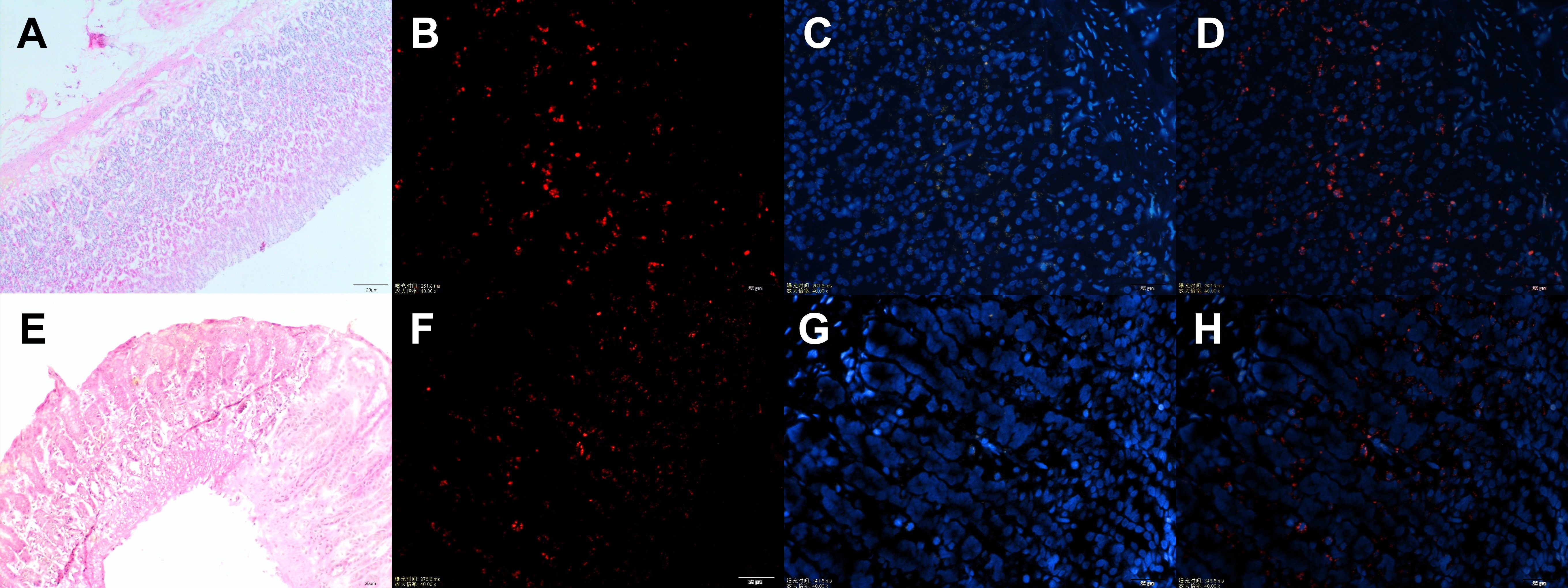
Injection of bone marrow-derived mesenchymal stem cells (BMMSCs)
could be colonized in gastric precancerous tissues. Representative images from
frozen pathological sections of rat gastric body (A–D) and gastric antrum
(E–H). (A,E) Hematoxylin and eosin (H&E)-stained image. (B,F) CM-Dil-labeled
BMMSCs in precancerous tissues. (C,G) DAPI nuclear staining. (D,H) Merged images. Exposure time, ms; magnification rate, 400
Using frozen pathological section, two antigens were labeled to identify epithelial and stromal cells, pan-keratin (Fig. 4A–D) and vimentin (Fig. 4E–H), respectively. The results revealed that some MSCs that colonized gastric tissues were differentiated into epithelial cells, while some MSCs retained the expression level of vimentin, an MSC marker.
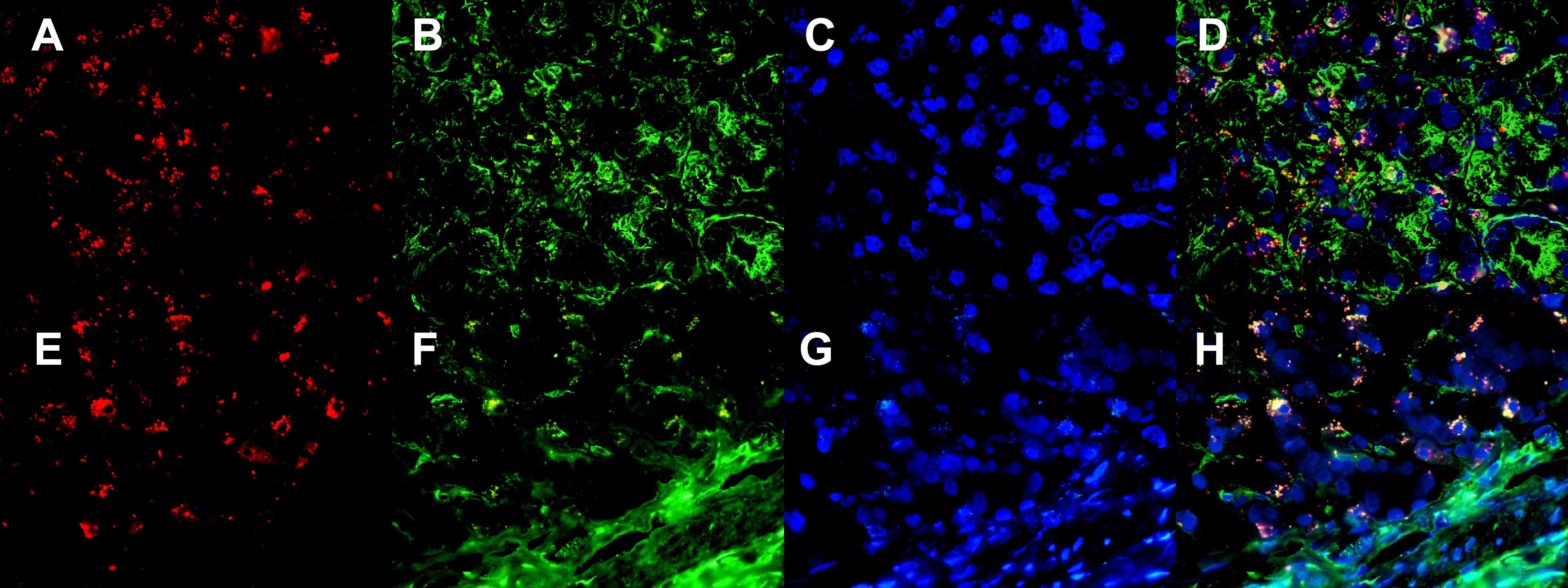
Bone marrow-derived mesenchymal stem cells (BMMSCs) were colonized in gastric precancerous lesions and differentiated into epithelial cells and mesenchymal cells. Frozen pathological section showing that BMMSCs colonized in gastric precancerous tissues could differentiate into epithelial cells (A–D) and mesenchymal cells (E–H). (A,E) CM-Dil-labeled BMMSCs. (B,F) Pan-keratin (B) and vimentin (F). (C,G) DAPI nuclear staining. (D,H) Merged images.
The growth of blood vessels can facilitate the repair of lesions. A low
expression level of VEGF was identified in normal gastric mucosa (Fig. 5A), and
the expression level of VEGF was significantly upregulated in gastric mucosal
cells within gastric lesions (Fig. 5B) compared with the normal group (p
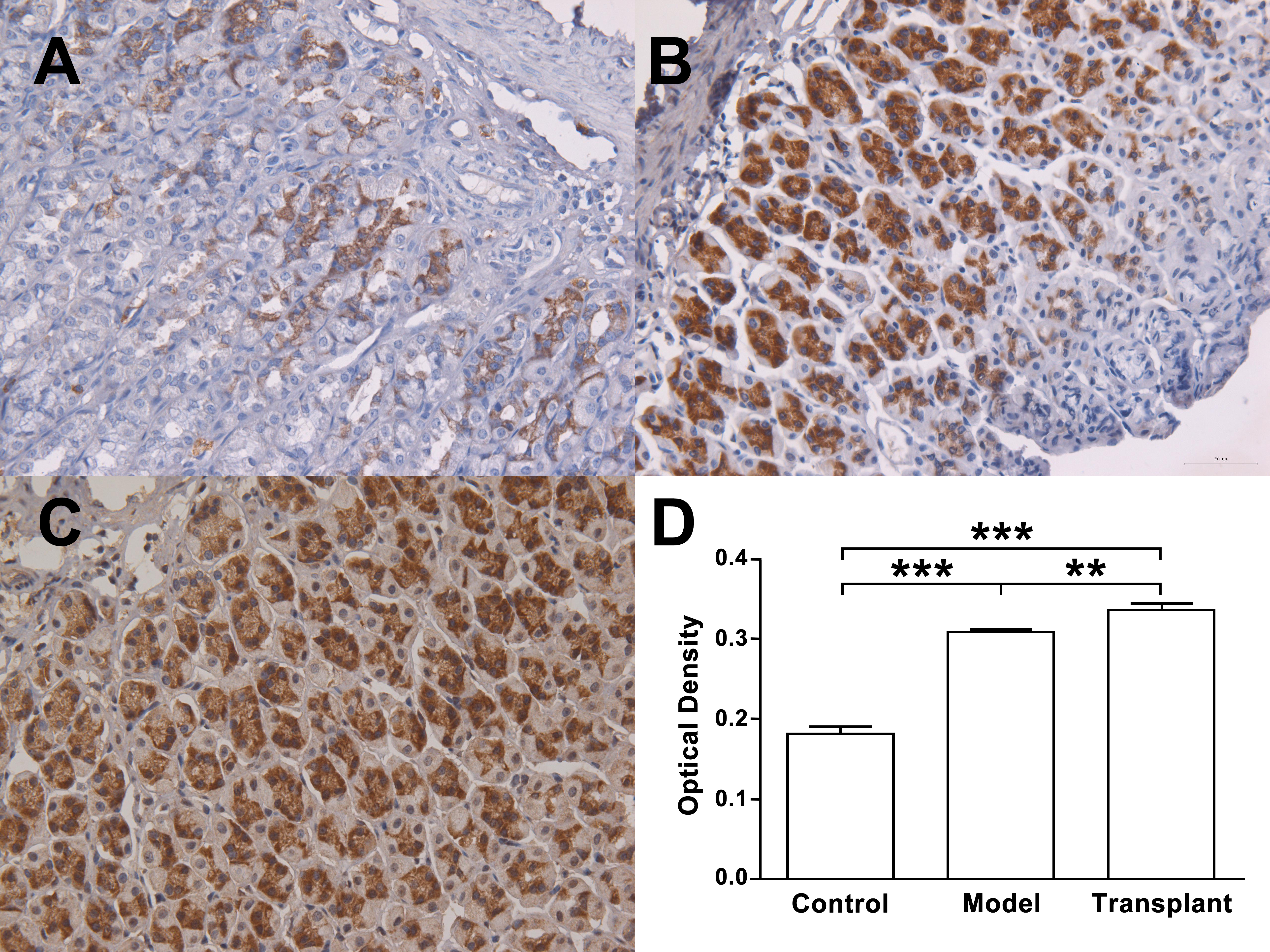
Bone marrow-derived mesenchymal stem cells (BMMSCs) promoted
vascular endothelial growth factor (VEGF) expression in gastric precancerous
tissue. (A–C) Immunohistochemical staining of frozen pathological sections from
normal, model, and BMMSC transplant groups. (A) Low VEGF expression in control
tissue. (B) Enhanced VEGF expression in model tissue. (C) Highest expression of
VEGF in transplant group. (D) Optical density was compared among the three
groups. n
Interleukins and interferons are important cytokines mediating the cellular
immune response and are closely associated with carcinogenesis. In the present
study, four cytokines potentially related to gastric carcinogenesis were selected
and their levels were measured in the serum of rats, including IL-4, IL-10,
IL-17, and IFN-
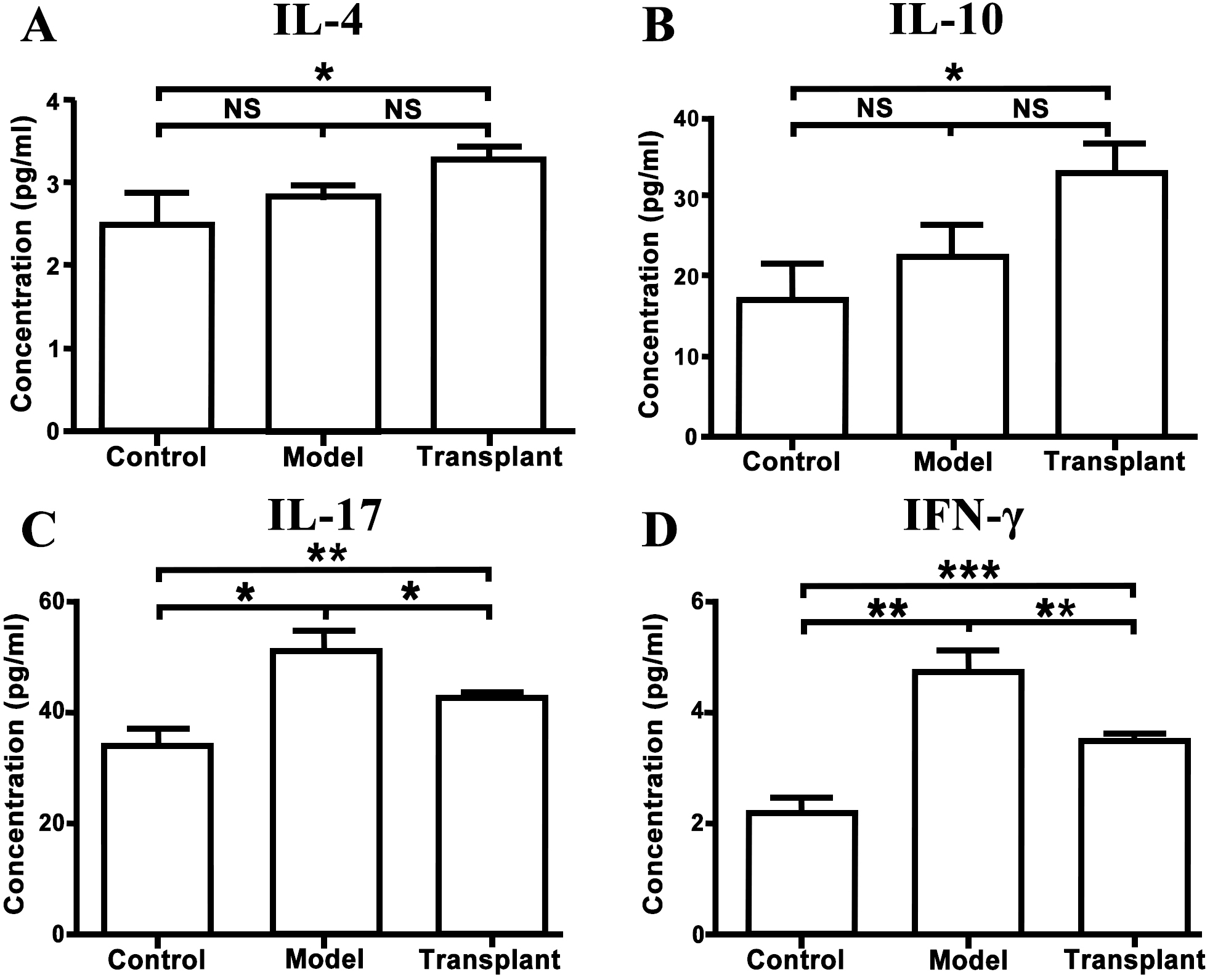
Bone marrow-derived mesenchymal stem cell (BMMSC)
transplantation regulated the serum levels of interleukins and interferon-gamma.
Serum levels of IL-4 (A), IL-10 (B), IL-17 (C), and IFN-
Group | IL-4 | IL-10 | IL-17 | IFN- |
Control | 2.53 |
17.33 |
34.30 |
2.20 |
Model | 2.82 |
22.73 |
51.23 |
4.76 |
Transplant | 3.28 |
33.33 |
42.84 |
3.48 |
In recent years, substantial progress has been achieved in both the research and practical application of MSCs. MSCs have been extensively employed in the investigation of various systemic diseases, such as myocardial infarction, Parkinson’s disease, inflammatory bowel disease, autoimmune diseases, diabetes, etc. They have emerged as highly promising seed cells for tissue engineering applications. Notably, multiple studies have demonstrated the in vitro differentiation capability of MSCs into gastrointestinal epithelial cells. Furthermore, there is evidence supporting the colonization of MSCs at sites of injured gastrointestinal mucosa, actively participating in the process of tissue repair [20, 21]. Local injection of MSCs was also found to facilitate the healing of gastric ulcers [22]. MSCs, when administered via the rat tail vein, are capable of circulating through the bloodstream and reaching various organs and tissues. In these locations, they actively engage in physiological regeneration and contribute to pathophysiological repair [23]. The colonization of MSCs is related to the transplantation method, time, frequency, and tissue microenvironment. When tissue injury is absent, the colonization of MSCs is correlated with the method of transplantation and undergoes redistribution over time. Notably, when administered through veins, MSCs exhibit the highest level of colonization in the lungs, followed by the kidneys, liver, and spleen. In contrast, the lowest level of colonization is found in gastrointestinal tissue [24]. In addition, local injection leads to tissue injury and MSCs are likely to die due to excessive local aggregation [17, 25]. The results of the present study revealed that, following MSC injection into the tail vein of rats, MSC colonization was observed in gastric lesions at the gastric body and antrum, while no colonization was found at gastric fundus. This could be attributed to the presence of squamous epithelium, scarce gastric glands, and insufficient blood supply.
Cytokeratin (CK) is one of the characteristic markers of epidermal and epithelial cells [26]. In the present study, it was revealed that MSCs could be differentiated into epithelial cells in vivo. Furthermore, it was observed that some MSCs retained the expression level of vimentin (an MSC marker). Migration to and colonization of sites of inflammation and tissue injury are characteristics of MSCs [27]. According to the findings of the present study, it is evident that inflammation plays a role in attracting MSCs to colonize gastric tissues in vivo. Subsequently, the colonized MSCs undergo differentiation into the appropriate target cells within the suitable local microenvironment. Therefore, it is noteworthy that the local microenvironment significantly influences the differentiation of MSCs into the required phenotypes following their migration.
BMMSCs display characteristic chemotaxis towards inflammation and sites of tissue damage, displaying the ability to home to sites of chronic inflammation. The local microenvironment can affect the directional differentiation of BMMSCs migrating to local tissues into cells with the correct phenotype, which is a prerequisite for their effectiveness. CK is a characteristic marker of epidermal or epithelial cells, and studies have demonstrated that BMMSCs can differentiate into epithelial cells in both in vitro and in vivo microenvironments [28, 29]. Typically, CK is not expressed by BMMSCs. The detection of CK expression level serves as an indicative marker, suggesting that BMMSCs may have undergone differentiation into epithelial cells. In the current study, pan keratin was used as an indicator of CK. Immunofluorescence assay revealed that staining of BMMSCs coincided with the staining for pan keratin. This indicates that colonized BMMSCs could differentiate into epithelial cells in the in vivo microenvironment. Vimentin is a type of intermediate filament protein that is mainly expressed in interstitial cells and nonepidermal cells. Tanaka et al. [30] and Hayashi et al. [31] demonstrated that in animal models, BMMSCs express vimentin as a mesenchymal cell marker, suggesting their capacity to differentiate into mesenchymal cells. In the present study, vimentin staining was observed in BMMSCs, indicating their ability to differentiate into mesenchymal cells under in vivo conditions. This finding aligns with existing literature reports.
Moreover, VEGF is a crucial soluble angiogenic factor with vascular-permeable properties [32]. It plays a vital role in enhancing microvascular permeability to mitigate harmful substances in the stomach, thereby safeguarding the gastric mucosa. VEGF achieves this by regulating the extracellular matrix, stimulating gland secretion, and promoting angiogenesis [33]. MSCs that colonize local tissue secrete VEGF, providing nutritional support to surrounding tissues and playing essential roles in inflammation and injury repair [32]. In the present study, a significant upregulation of VEGF was found in the gastric mucosal tissue in both the model and MSC transplant groups compared with the control group. Notably, the expression of VEGF in the transplant group was significantly higher than that in the model group. These findings suggest that gastric mucosal injury and lesions induced stress and repair responses, stimulating local VEGF expression. This increase in VEGF expression could potentially enhance vascular permeability and gastric mucosal blood flow. MSCs, with the capability of migration to inflammatory lesions, further promoted VEGF production within gastric tissues, contributing to tissue repair and defense by improving the vascular microenvironment.
MSCs are stem cells with low immunogenicity, and they play pivotal roles in
regulating immunity. Transplanted MSCs can inhibit the activation of T cells
[12], reduce local inflammation, and provide a favorable environment for tissue
repair [34]. The immunomodulatory activity of MSC is closely associated with
IFN-
In present study, it was found that the serum concentrations of IL-17 and
IFN-
In summary, MSC transplantation significantly attenuated the inflammatory response in a rat model of gastric lesions. Both morphological and histological observations revealed that MSC transplantation could contribute to the recovery of gastric lesions. Transplantation not only reverses lesions and diminishes inflammation, but also holds promise for potential therapeutic effects on gastric diseases. BMMSCs may be ideal cells for stem cell therapy to inhibit the progression of gastric lesions by anti-inflammatory and immunomodulatory mechanisms.
Firstly, the occurrence and development of tumors are protracted processes. Due to constraints in experimental duration, our observation of BMMSC colonization was limited to a relatively short period. Consequently, we were unable to scrutinize the enduring consequences of the interaction between the local microenvironment and BMMSCs over the long term. Secondly, the mechanism by which BMMSCs intervene in gastric precancerous lesions warrants further research.
GC, gastric cancer; MSCs, mesenchymal stem cells; BMMSCs, bone marrow-derived
mesenchymal stem cells; BM, bone marrow; UC, ulcerative colitis; DMEM, Dulbecco’s
modified Eagle’s medium; MNNG, N-methyl-N
The datasets used and/or analysed during the current study are available from the corresponding author on reasonable request.
QC: Original draft, Formal analysis; CW: Writing — review & editing, Data curation; WW: Resources, Software; YG: Conceptualization, Methodology, Supervision; HC: Conceptualization. All authors contributed to editorial changes in the manuscript. All authors read and approved the final manuscript. All authors have participated sufficiently in the work and agreed to be accountable for all aspects of the work.
All experiments were approved by the Ethics Committee of the Animal Facility of Chinese PLA General Hospital, and were conducted in conformance with the guidelines for caring for laboratory animals.
We thank Medjaden Inc. for its assistance in the preparation of this manuscript.
This study was supported by grants from the National Natural Science Foundation of China (No. 82271628).
The authors declare no conflict of interest.
Publisher’s Note: IMR Press stays neutral with regard to jurisdictional claims in published maps and institutional affiliations.