- Academic Editor
Background: Chronic pain refers to pain that persists for over three
months. Chronic pain may restrict activities of daily living, including work,
learning, social life, and can lead to anxiety, depression, and sleep
disturbance. Imaging data have demonstrated that central sensitization often
occurs in the brain of patients with chronic pain, which arises from imbalanced
neurotransmission in the central nervous system. Transient receptor potential
vanilloid 1 (TRPV1) is an ion channel to serve as an inflammatory detector in the
brain. We aim to determine the properties of acupoint catgut embedding (ACE) on
cold stress-induced mice fibromyalgia (FM) and surveyed the character of TRPV1
and linked molecules in chronic FM pain. Methods: Intermittent cold
stress (ICS) was used to induce mice FM model. Mice were subgrouped into normal
mice, ICS-induced FM group, FM mice with ACE, and FM in Trpv1
Acute pain typically accompanies injury, such as wounds, tissue damage, or
fractures. Various pharmacological agents are available to relieve acute pain. In
contrast, chronic pain persists long after recovery from the injury or damage.
About 1/4 of adults suffer from chronic pain, identified as discomfort sustained
for over three months, and accounts for a high proportion of insurance claims
[1]. Chronic pain can result from various etiologies including arthritis, cancer
pain, low back pain, headaches, muscle pain, and fibromyalgia (FM). FM is
presently described as a complex disease characterized by chronic widespread
muscle pain, fatigue, insomnia, anxiety, and depression [2]. FM is a growing
issue affecting individuals, insurance providers, healthcare systems, economies,
and societies. There are no curative therapies for FM and methods to relieve FM
pain are lacking due to its unclear fundamental mechanisms. The occurrence of FM
is around 2–8% of people, depending on the analytic principles used [2]. New
diagnostic principles for FM to include widespread pain index (WPI) and symptom
severity scale (SS). The WPI examines 19 common body pain parts for two weeks.
The SS calculates the degree of fatigue, waking, and cognitive symptoms. FM is
characterized as WPI
Acupuncture is an ancient Chinese medicine technique that involves implanting a steel needle into an acupoint. Acupuncture is helpful in managing FM due to its ability to reduce pain, anxiety, depression, and insomnia [6, 7]. In addition, electroacupuncture (EA) has been described to attenuate numerous kinds of pain [8, 9, 10, 11, 12]. Recently, acupoint catgut embedding (ACE) was developed as a novel technique to establish constant acupoint stimulation. ACE treatment has been shown to be more effective and its benefits prolonged than acupuncture or EA in managing obesity [13]. ACE has the advantages of long-term effects and low cost, and has been broadly utilized in the management of acute or chronic pain [14, 15]. Recent articles showed that ACE activates the immune system because the absorbable chromic catgut sutures are recognized as a non-self protein [16]. ACE can induce long-term immune responses such as macrophage phagocytosis and neutrophil infiltration [17]. ACE can also reliably diminish postoperative pain and decrease analgesic medicine intake [18].
Transient Receptor Potential Vanilloid (TRPV) is an ion channel chiefly located
on the neuronal membrane. TRPV can be activated by vanilloid compounds and has
six subtypes. TRPV1 was first recognized as a receptor of capsaicin, the major
constituent of chili pepper [19]. TRPV1 exists in the dorsal root ganglion,
spinal cord, and trigeminal ganglion for pain sensation and transmission. In
peripheral regions, TRPV1 greatly exists in A- and C-type sensory neurons to
detect inflammation and painful stimuli. TRPV1 was also described to exists in
the central nerve system (CNS) for thermoregulation, synaptic transmission, and detection of
inflammation [19]. Furthermore, TRPV1 is expressed in non-neuronal astrocytes or
glial cells for pain signaling [20]. TRPV1 is expressed in several brain areas
including thalamus, somatosensory cortex, prefrontal cortex, hippocampus,
amygdala, hypothalamus (Hypo), and cerebellum (CB). TRPV1 activation sequentially
activates kinases, such as protein kinase A (PKA), phosphoinositide 3-kinases
(PI3K), protein kinase C (PKC), and Ca
The plan of the current study was to verify our hypotheses that FM is related to augmenting TRPV1 signaling in the mouse brain and that ACE ameliorates both mechanical and thermal nociception through suppression of TRPV1 pathway. To address these questions, we used a mouse FM through intermittent cold stress (ICS). Consistent with our hypothesis, mice subjected to ICS exhibited significant chronic mechanical and thermal pain that could be abridged by ACE or TRPV1 gene deletion. TRPV1 pathway was amplified in the mouse Hypo and CB and can be relieved by ACE and TRPV1 gene loss. These data suggest the therapeutic effect of ACE is connected with TRPV1 in the mouse brain. We propose that the TRPV1 signaling pathway is a potential target for FM treatment.
The treatment of mice was permitted by China Medical University Committee
(CMUIACUC-2022-424), Taiwan, subsequent the guideline for the practice of
experimental animals (National Academy Press). The current work is indicated
according to the ARRIVE guidelines. Totally, 40 female mice were used in the
current study, containing 30 normal mice (BioLASCO, Co., Ltd, Taipei, Taiwan) and
10 Trpv1
All animals were accommodated at 24
ACE mice had received ACE treatment at the bilateral stomach 36 (ST36) acupoint
at days 0 and 7. Like human anatomy, the ST36 acupoint is sited longitudinally at
3 mm under the apex of patella bone intersecting horizontally 1 mm lateral to
anterior the tibia bone at the central of the tibialis anterior muscle.
Sterilized needle 0.6
Mechanical or thermal nociceptive performances were measured 7 periods from day
0 to 14 after FM induction. We first verified the von Frey filament examination,
mechanical pain was tested by calculating the strength of replies to stimuli
within 3 performances of von Frey test (IITC Life Science Inc, Los Angeles, CA,
USA). Animals were then placed to a steel net (75
Animals were sedated under isoflurane and suffered cervical dislocation. Mice
Hypo and CB samples were separated to draw out proteins and further kept at –80
°C refrigerator for protein mining. All extracted samples were
standardized with radioimmunoprecipitation (RIPA) with: 1% NP-40,
50 mM Tris-HCl, 0.02% NaN
Animals had been anaesthetized with isoflurane and intracardially filled with
0.9% normal saline and then 4% paraformaldehyde. The mice brain was instantly
cut apart and next fixed with 4% paraformaldehyde in refrigerator for 2–3 days.
The brains were next engaged in 30% sucrose in 4 °C
refrigerator for 2–3 days. The mice tissue was fixed with optimal cutting
temperature compound and quickly stored at –80 °C
refrigerator. Mice brains were sliced and then placed on glass slides (20
µm). The tissues were further rinsed with 3% BSA, 0.1% Triton X-100, and
0.02% NaN
Statistical differences were accomplished by using the SPSS software (SPSS 21,
IBM Corp., Chicago, IL, USA). All results are offered as the mean with standard
error (SEM). Shapiro-Wilk examination was achieved to examination the familiarity
of results. Statistical differences in four groups were confirmed via the
analysis of variance (ANOVA) test and a post hoc Tukey’s test. p
We induced a mice FM and determined their analgesic outcome of ACE and
TRPV1 gene deletion. All mice showed similar mechanical threshold
tendencies, which was normally distributed at baseline and was not statistically
different among all groups. Cold stress reliably induced mechanical hyperalgesia
(Fig. 1A, red circle, D14: 1.96
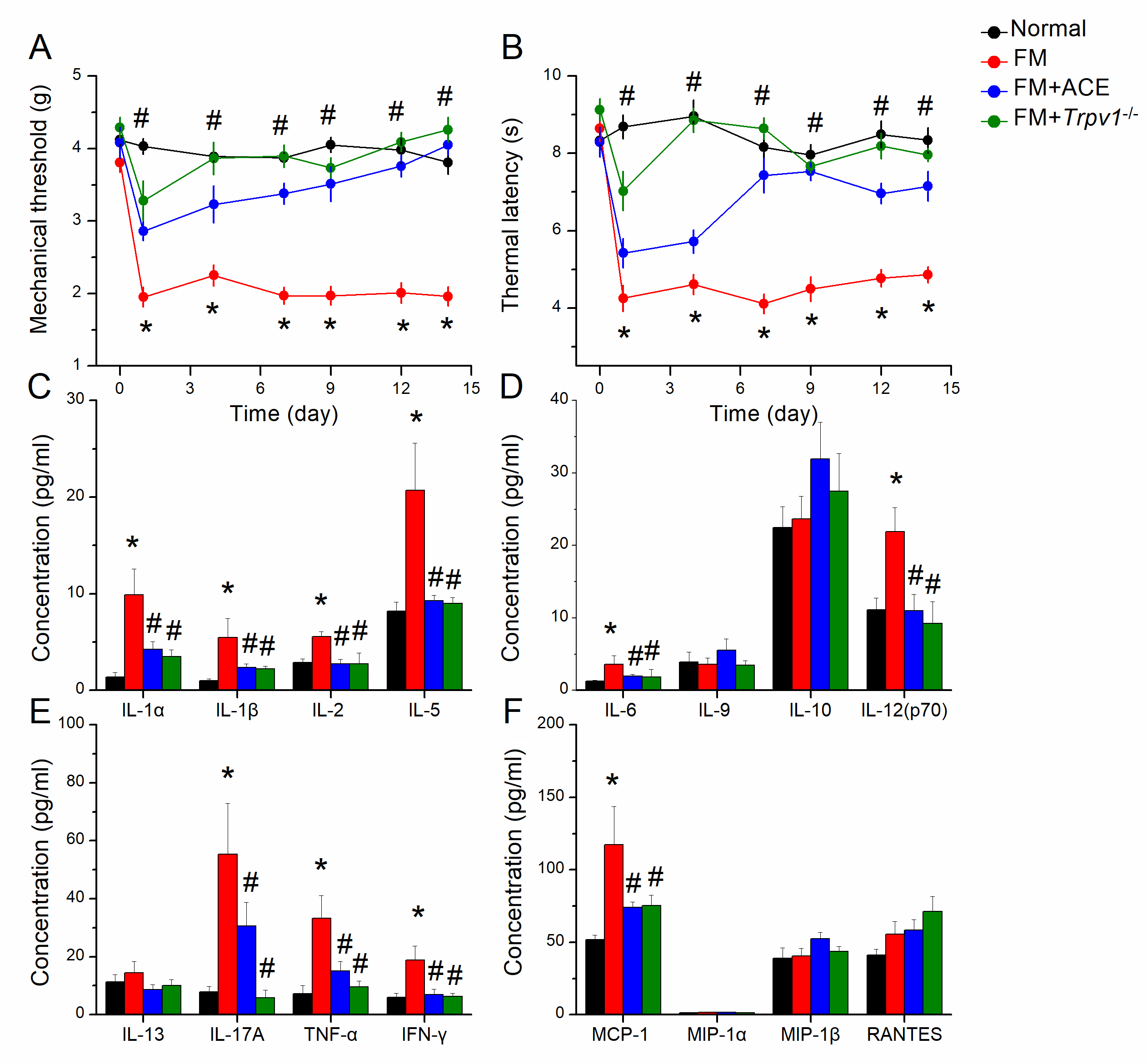
Mechanical, thermal pain, and inflammatory cytokines in normal,
FM, FM+ACE, and FM+Trpv1
Neuroinflammation was detected in the blood and cerebral spinal fluid in FM
patients. Accordingly, we determined the concentration of inflammatory cytokines
in mouse plasma through multiplex ELISA technique. We measured interleukin-1
alpha (IL-1
Hypothalamic dysfunction was reported in FM patients. We performed Western blot
to count the protein intensities of molecules in the TRPV1 in the mice
hypothalamus. The level of TRPV1 was detected in mice hypothalamus and increased
in FM mice (Fig. 2A, black column, * p
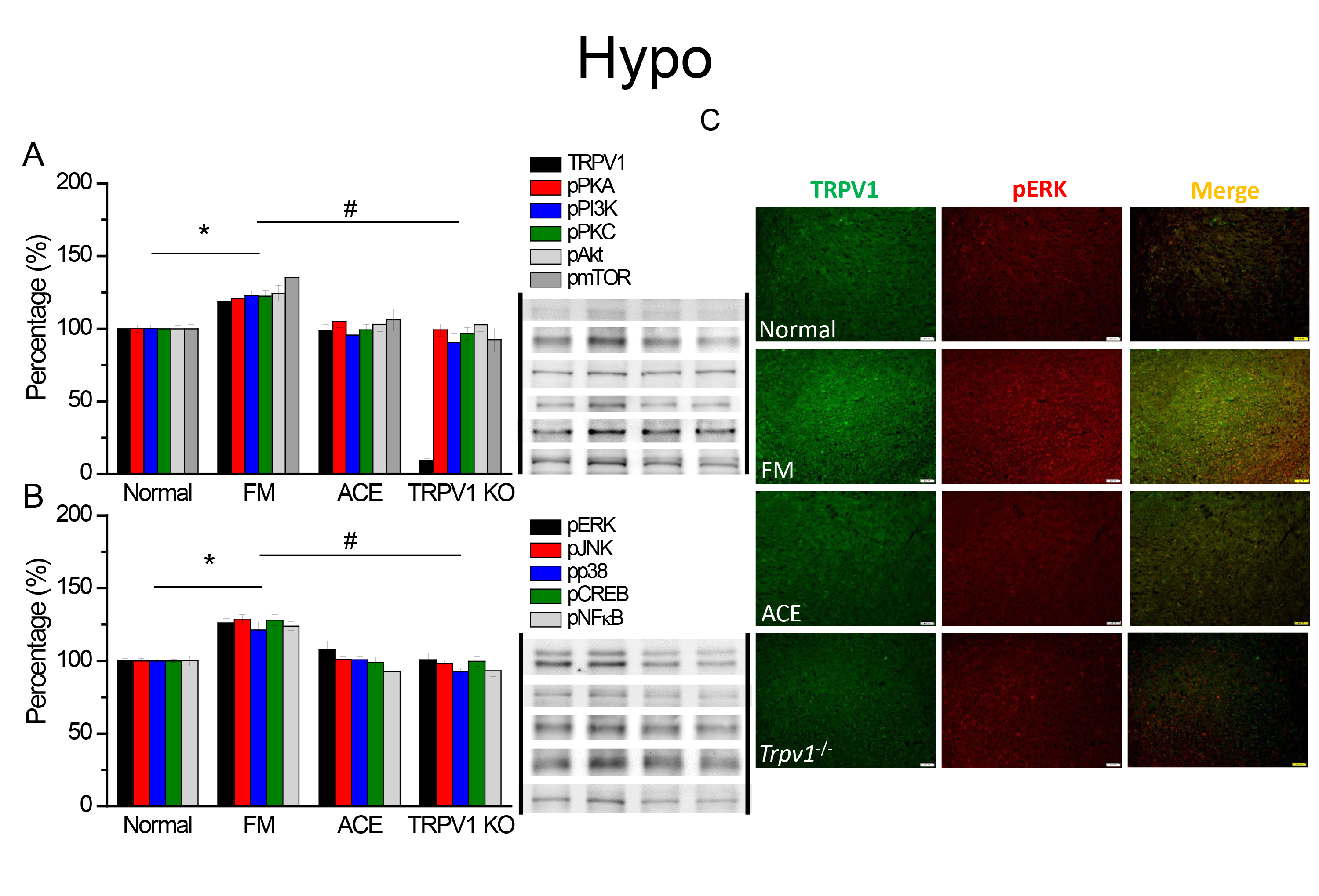
TRPV1 and linked kinases in the mice hypothalamus. The western
blot signals of Normal, FM, ACE, and Trpv1
We next used immunofluorescence staining to measure TRPV1 in the hypothalamus.
We detected low levels of TRPV1 in normal mice and increased levels in FM mouse
hypothalamus (Fig. 2C, green color, n = 4). ACE and TRPV1 loss consistently
abolished the augmentation of TRPV1 (Fig. 2C, green color, n = 4). A similar
tendency was also observed in pERK appearance (Fig. 2C, red color, n = 4). TRPV1
and pERK colocalization increased in the FM mice but was diminished in ACE and
Trpv1
We investigated protein levels in the CB5. TRPV1 protein was significantly
augmented after FM induction (Fig. 3A, * p
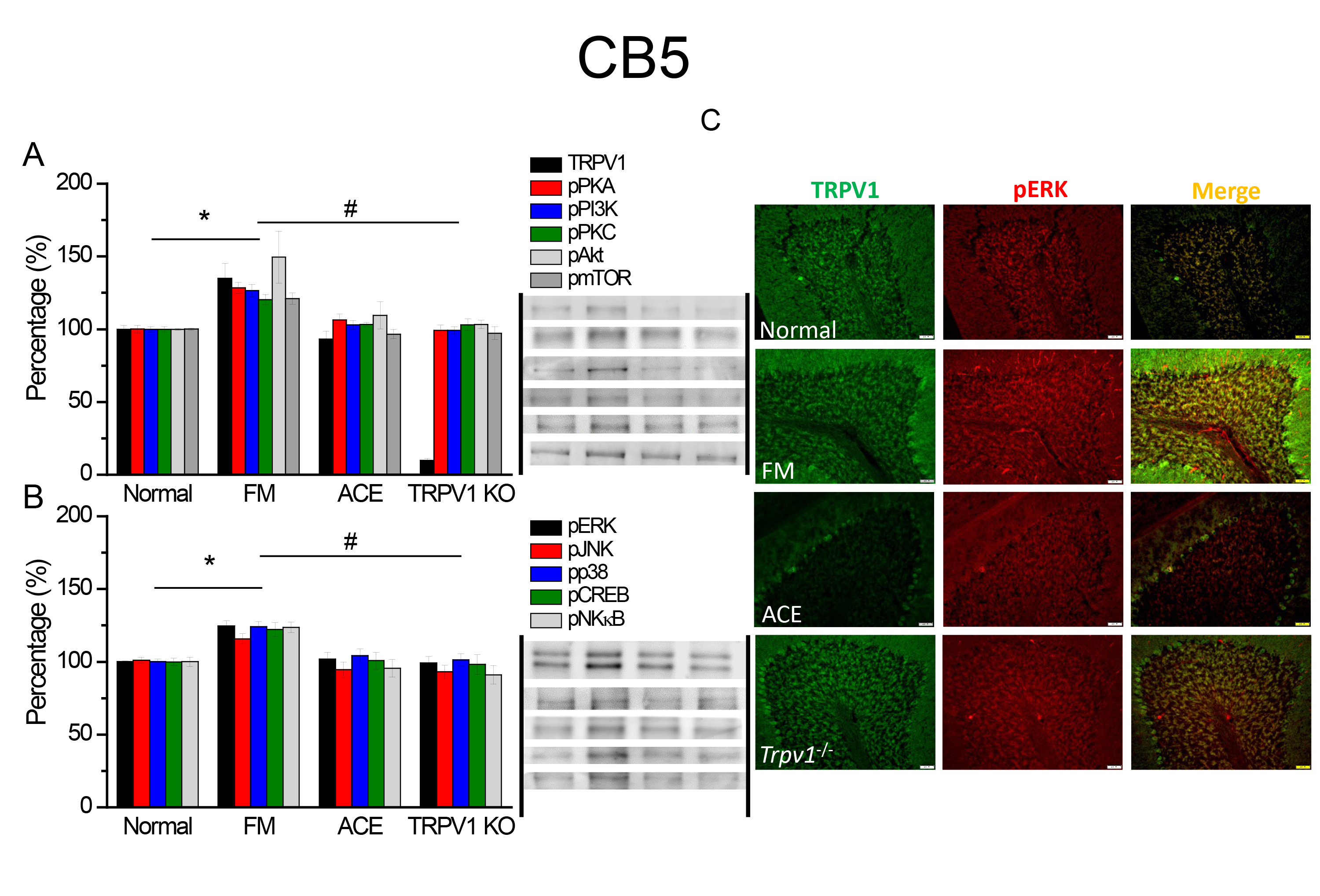
The intensities of TRPV1 and associated factors in CB5. The
western blot signals of Normal, FM, FM+ACE, and Trpv1
Our data determined the TRPV1 and correlated kinases in the CB6 and CB7 in mice.
TRPV1 proteins were significantly augmented after FM stimulation, compared with
normal mice (Figs. 4,5A, black column, * p
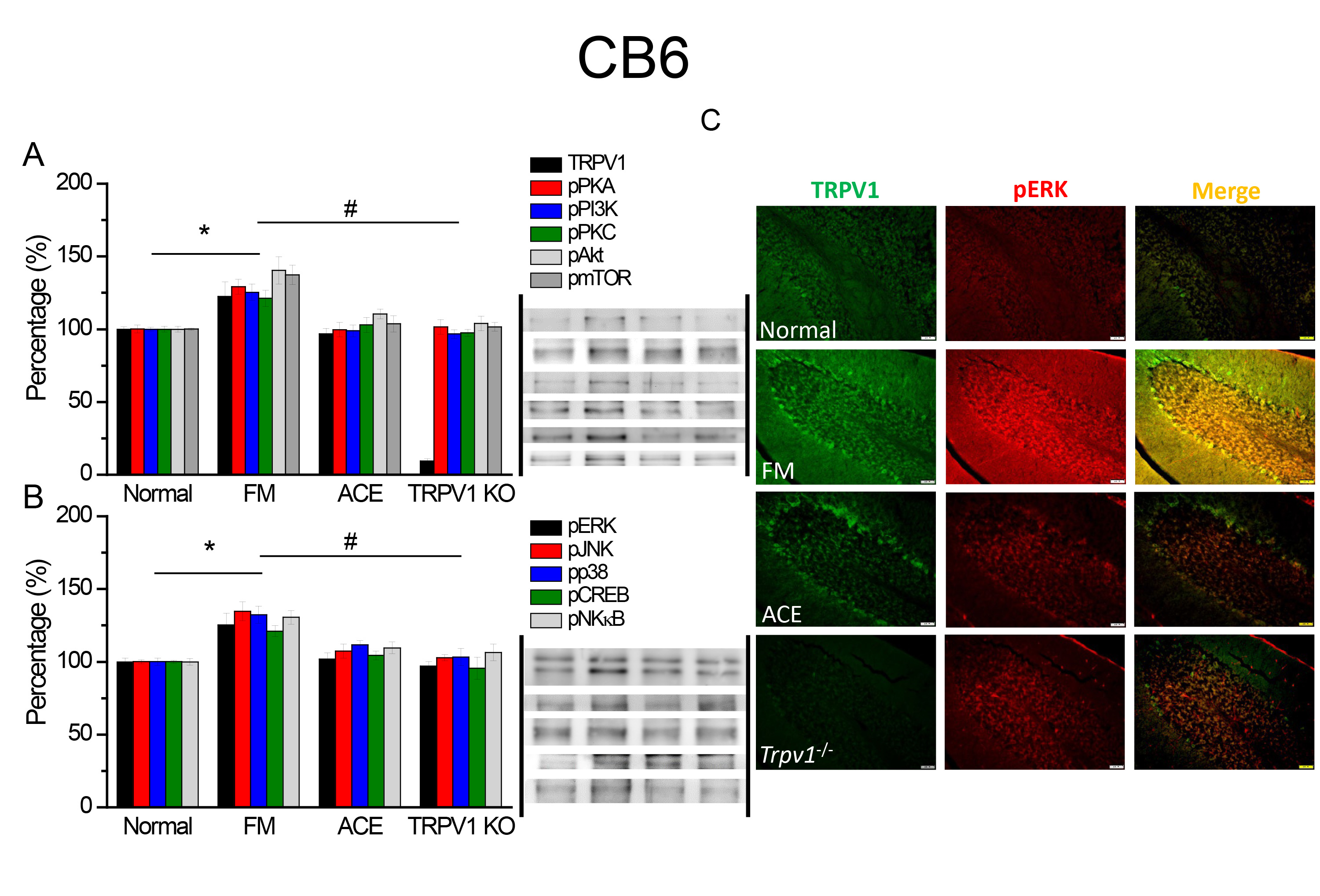
TRPV1 and associated kinases in CB6. The western blot signals
of Normal, FM, FM+ACE, and Trpv1
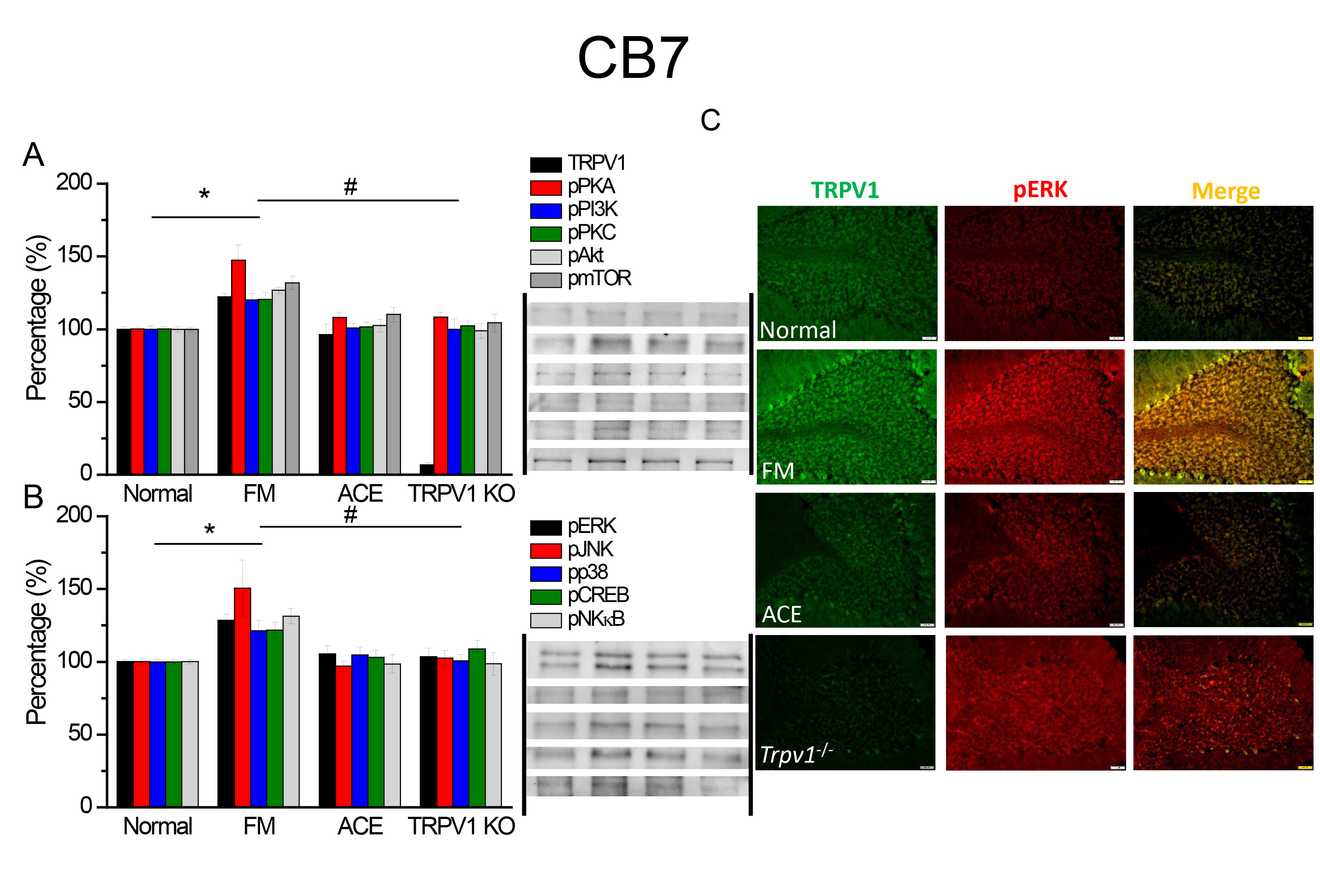
TRPV1 and associated kinases in CB7. The western blot signals
of Normal, FM, FM+ACE, and Trpv1
We used immunofluorescence to determine the localization of TRPV1 and pERK in
CB6 and CB7 following FM induction. TRPV1 levels were significantly increased in
the CB6 and CB7 in FM mice (Figs. 4,5C, green fluorescence, n = 4), while the
result was diminished in the ACE and Trpv1
We reveal that the FM model mimics the clinical symptoms of chronic mechanical and thermal nociception in FM mice. We then presented that ACE at ST36 site reliably attenuated either mechanical or thermal pain. Similar decreases in pain-related behaviors were also found in mice lacking TRPV1 receptors. TRPV1 and related kinases were all increased in the mice Hypo and CB, which are brain areas involved in pain modulation. These molecules were sequentially diminished by ACE and TRPV1 gene deletion, signifying that the therapeutic effect of ACE is linked with TRPV1 in the mice Hypo and CB. Our data provide suggestion that TRPV1 signaling pathway molecules could potentially be beneficial targets for FM treatment.
Peripheral nerve stimulation (PNS) is extensively used in several clinical scenarios, especially for pain control. ACE shares similar effects as PNS and transcutaneous electrical nerve stimulation for chronic pain management. PNS is utilized in several chronic pain situations, such as peripheral nerve injury, local pain syndrome, and FM [24, 25]. Gilmore et al. [26] reported that chronic neuropathic pain is an underdiagnosed nociceptive condition following elimination. They verified the possibility of inserting fine-wire PNS inserts into the sciatic and femoral nerves for pain control. They suggest that PNS may deliver noteworthy pain assistance and facilitate recovery in chronic neuropathic pain patients. They also showed that 60-day PNS stimulation achieves continuous control of chronic pain [26]. A recent article showed that PNS works through both peripheral and central mechanisms. In its central mechanism, PNS can activate dorsal horn interneurons to block pain signals, attenuating central sensitization and hyperalgesia. In the spinal cord, PNS may increase the release of neurotransmitters, including Gamma-aminobutyric acid (GABA), glycine, serotonin, and cannabinoid pathways [27]. PNS in peripheral sites can attenuate nociceptive afferent signals via changes in the local microenvironment, decrease in neurotransmitters and inflammatory cytokines, and frequency of neural discharge [28, 29].
Cui et al. [30] showed that ACE can alleviate the overexpression of N-methyl-D-aspartate receptor (NMDA), pCaMKII, pERK, and pCREB by CFA-treated rats. The shot of the serotonin 1A receptor agonist mimicked the properties of ACE and was further blocked by the serotonin 1A receptor blocker WAY-100635 [30]. Du et al. [31] reported that ACE has analgesic effects measured as withdrawal thresholds and can decrease paw edema in a mouse inflammatory pain model. ACE markedly ameliorated the increased protein level of the sigma receptor in the mouse lumbar spinal cord. ACE also attenuated the increase in pERK and pp38, and injection of the sigma receptor agonist PRE084 significantly reversed the phenomena [31]. Our recent publications show that EA can relieve mice FM pain through TRPV1 pathway [8, 10]. The current study further determined that ACE has an analgesic effect through suppression of TRPV1 activity similar to EA. These results indicate the beneficial effect of ACE for continuous and prolonged treatment for FM.
Recently, we showed that EA can relieve both acute and chronic pain through the TRPV1 pathway. In this study, we provide evidence that cold stress chronic pain may be associated with increased TRPV1 in the mouse brain and that ACE exerts its analgesic effect via suppression of TRPV1 activity in these areas. To address this issue, we induced chronic pain by cold stress and observed pain-related behaviors in mice. Consistent with our hypothesis, cold stress meaningfully convinced mechanical and thermal hyperalgesia, and ACE reliably reversed this nociception. In addition, chronic pain induction increased TRPV1 signaling pathway and correlated kinases in the mouse Hypo and CB. Furthermore, ACE and TRPV1 gene deletion dramatically reduced these molecular changes, suggesting that TRPV1-induced central sensitization can be reversed.
We demonstrate that the antinociceptive effect of ACE is dependent on TRPV1 pathway in the mouse brain and that TRPV1 signaling pathways participated in pain control in the FM model (Fig. 6). We thereby propose that these could be possible novel targets for the management of chronic pain.
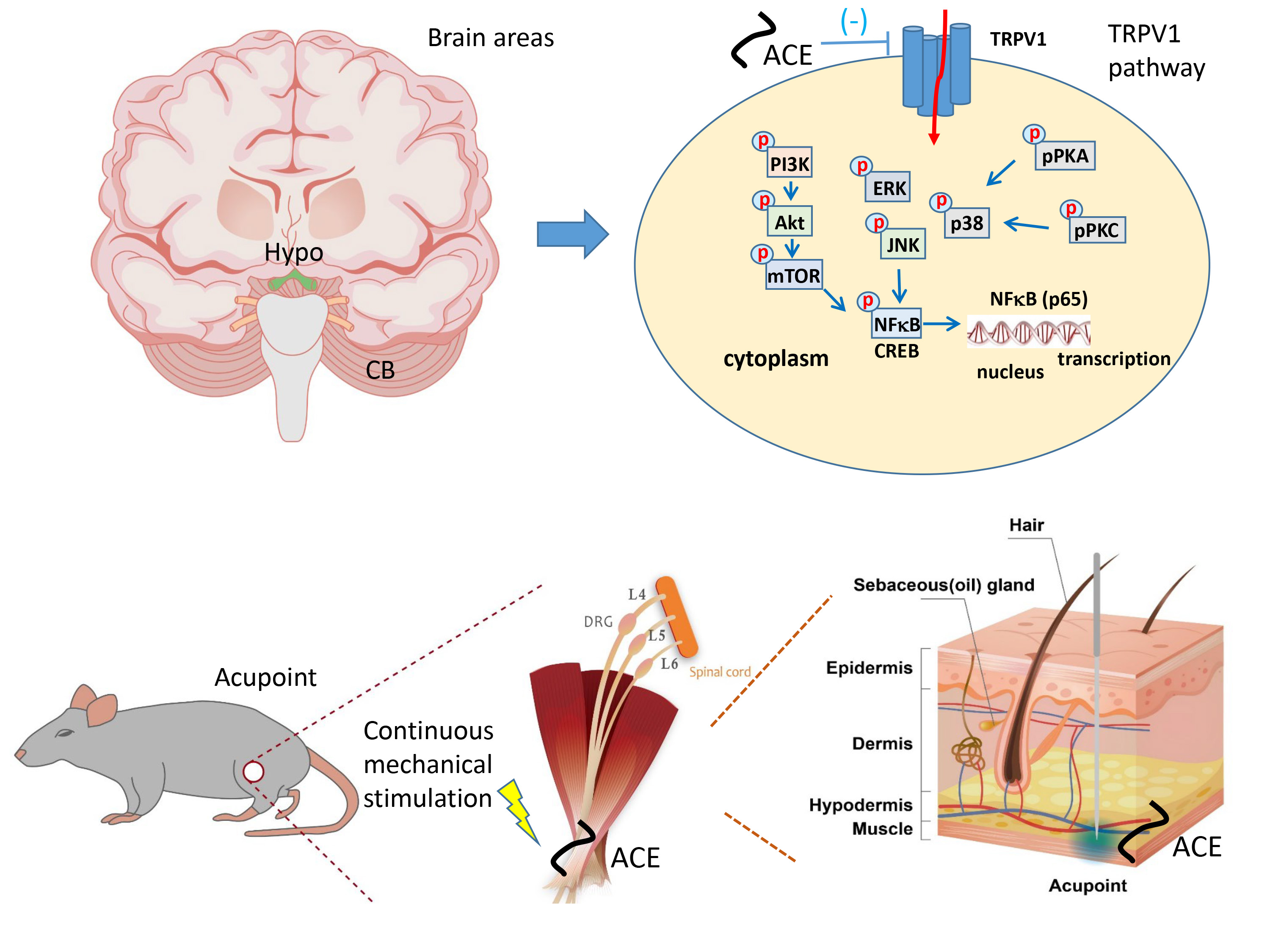
Schematic diagram of TRPV1 and related factors altered by ACE.
The summary picture shows the crucial effect of analgesic mechanisms involving
TRPV1 and related factors in mice FM pain. ACE or Trpv1
CNS, central nervous system; ACE, Acupoint catgut embedding; TRPV1, Transient
receptor potential vanilloid 1; FM, fibromyalgia; ICS, intermittent cold stress;
WPI, widespread pain index; SS, symptom severity scale; Hypo, hypothalamus; CB,
cerebellum; PKA, protein kinase A; PI3K, phosphoinositide 3-kinases; CaMKII,
Ca
The data used to support the findings of this study are available from the corresponding author upon request.
PCL, CMY, and IHH performed the experiments, analyzed and interpreted the data, and wrote the draft manuscript. YHC advice on the Western blot and ELISA experiments. YWL analyzed the data and draw the figures. YHC and YWL designed the research study and revised and submitted the manuscript. All authors contributed to editorial changes in the manuscript. All authors read and approved the final manuscript. All authors have participated sufficiently in the work and agreed to be accountable for all aspects of the work.
The treatment of mice was permitted by China Medical University Committee (CMUIACUC-2022-424), Taiwan, subsequent the guideline for the practice of experimental animals (National Academy Press).
The authors would like to thank Enago (https://www.enago.tw/) for the English language review.
This research was funded by Tzu Chi University TTCRD112-14, China Medical University CMU111-S-25, and the “Chinese Medicine Research Center, China Medical University” from The Featured Areas Research Center Program within the framework of the Higher Education Sprout Project by the Ministry of Education (MOE) in Taiwan.
The authors declare no conflict of interest.
Publisher’s Note: IMR Press stays neutral with regard to jurisdictional claims in published maps and institutional affiliations.