- Academic Editor
Background: Periodontitis is one of the most common chronic inflammatory disorders in adults. Although clinical studies have suggested a causal relationship between periodontitis and major depression (MD), the biological mechanisms by which periodontitis instigates MD are unknown. We investigated whether a systemic administration of lipopolysaccharide (LPS) from Porphyromonas gingivalis (Pg), a major Gram-negative pathogen of periodontitis, causes depressive-like behavior and glial activation in the hippocampus and the prefrontal cortex (PFC), which are MD-related brain regions. Materials and Methods: Eight-week-old male Sprague Dawley rats were randomly divided into a behavioral test group and an immunohistochemistry group. The rats in each group were further assigned to the sham injection (saline) and Porphyromonas gingivalis-lipopolysaccharide (Pg-LPS) injection protocols. The rats received an intraperitoneal injection of saline or Pg-LPS with gradually increasing doses (day 1: 0.5, day 2: 0.5, day 3: 0.75, day 4: 0.75, day 5: 1.0, day 6: 1.0, and day 7: 1.0 mg/kg of body weight) for seven consecutive days. After the systemic administration, the behavior test group underwent the forced swimming test (FST) and Y-maze test. For the immunohistochemistry group, we quantified the immunoreactivity for microglial Iba-1 (ionized calcium-binding adapter molecule 1) and astrocytic glial fibrillary acidic protein (GFAP) in the hippocampus (dentate gyrus [DG], cornu ammonis [CA1 and CA3]) and PFC (prelimbic [PrL] and the infralimbic [IL]) areas. Results: The FST immobility time in the Pg-LPS group was significantly longer than that in the sham group. In the Y-maze test, a significant decline in spontaneous alternation behavior was observed in the Pg-LPS group compared to the sham group. The peripheral administration of Pg-LPS significantly increased the immunoreactivity for Iba-1 in the CA3 and PrL. Pg-LPS injection significantly increased the immunoreactivity for GFAP in the DG, CA1, and CA3. Conclusions: The major result of this study is that a repeated systemic administration of Pg-LPS caused depressive-like behavior and both microglial and astrocytic activation in rats. This finding may comprise biological evidence of a causal relationship between periodontitis and MD.
Major depression (MD) is a prevalent life-threatening psychiatric disorder. Approximately 322 million people are affected by MD worldwide [1]. The neuroinflammatory process in which activated microglia and astrocytes generate excessive pro-inflammatory cytokines has been implicated in the pathogenesis of MD [2, 3, 4]. Increasing evidence also suggests that infection and persistent low-grade inflammation in peripheral tissues play a role in MD [5]. Periodontitis is one of the most common chronic inflammatory diseases in adults [6, 7, 8]. Although recent systematic reviews and meta-analyses have suggested a mutual relationship between periodontitis and MD [9, 10, 11, 12, 13], the biological mechanisms by which periodontitis instigates MD are unclear.
Several animal studies have demonstrated that peripheral administration of lipopolysaccharide (LPS) from Escherichia coli increases the levels of pro-inflammatory cytokines in both the periphery and brain and induces abnormal behavior similar to MD, called depressive-like behavior [14, 15, 16, 17]. It has also been believed that systemic inflammation causes the neuroinflammatory process through several pathways [5]. It is proposed that such neuroinflammation, in turn, elicits MD symptoms by influencing brain functions, especially neurotransmitter metabolism [18]. We conducted the present study to determine whether repeated systemic inflammation induced by intraperitoneal (i.p.) injection of LPS from Porphyromonas gingivalis (Pg), a major Gram-negative pathogen associated with periodontitis, causes depressive-like behavior and glial activation in the rat hippocampus and the prefrontal cortex (PFC), which are MD-related brain regions. Previous rodent studies along this line have demonstrated that i.p. injection of Porphyromonas gingivalis-lipopolysaccharide (Pg-LPS) causes depressive-like behavior [19] and cognitive dysfunction [20] with glial activation.
Eight-week-old male Sprague Dawley (SD) rats (SLC, Inc., Shizuoka, Japan) were
used. They were housed in plastic cages at a room temperature of 23
Pg-LPS was purchased from InvivoGen (catalog no. tlrl-pglps, version no. 14F18-MM, San Diego, CA, USA). Pg-LPS was dissolved in endotoxin-free water.
We randomly divided the SD rats into a behavioral test group and an immunohistochemistry group. Each group was further assigned to the sham injection and Pg-LPS injection groups. It has been documented that the stress intensity of the forced swimming test (FST) is very strong [21], and the FST stress has the possibility of affecting brain structure and function [22]. In order to avoid such influence on immunohistochemistry results, we divided rats into the group undergoing behavior tests only and the group undergoing the immunohistochemical analysis only. The number of individuals in each group was 5 or 6. Investigators were not blinded to the group allocation at any stage.
The rats in the Pg-LPS subgroup received Pg-LPS at gradually increasing doses by daily i.p. injection for seven consecutive days (Fig. 1). The Pg-LPS dose was increased as follows: day 1: 0.5, day 2: 0.5, day 3: 0.75, day 4: 0.75, day 5: 1.0, day 6: 1.0, and day 7: 1.0 (mg/kg of body weight (BW)). Repeated daily administration of increasing LPS doses has been shown to induce depressive-like behavior more robustly, compared to administration of consistent LPS dose, with avoidance of LPS tolerance [15].
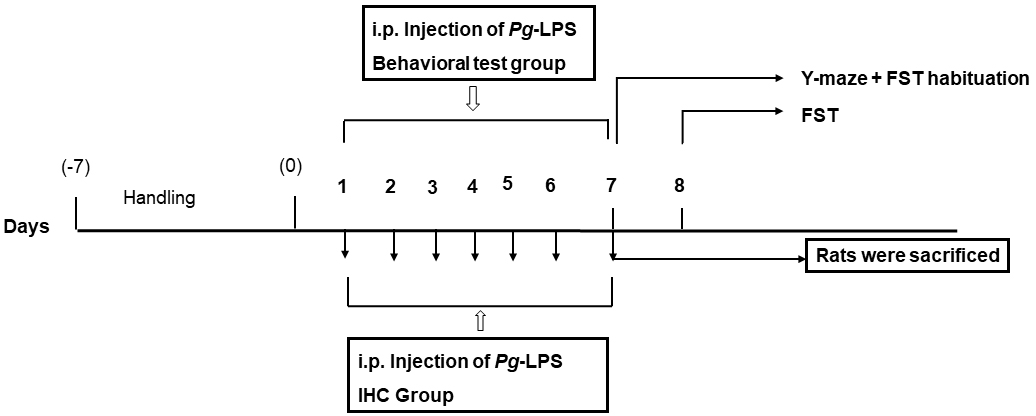
The experimental schedule. Pg, Porphyromonas gingivalis; LPS, Lipopolysaccharide; i.p., intraperitoneal; IHC, immunohistochemistry; FST, forced swimming test.
The sham injection group received an equivalent volume of saline intraperitoneally and daily for seven consecutive days. In the behavioral test group, the Y-maze test was performed 2 hr after the last i.p. injection. 2 hr after the Y-maze test, habituation of the forced swimming test (FST) was carried out. In the immunohistochemistry group, brain samples were collected 2 hr after the last injection (Fig. 1).
The forced swimming test (FST) was performed as described [23]. Briefly, the rat was placed in a cylinder of water for 6 min, and its behavior was recorded by a video camera. The time that the rat spent in an immobile state (i.e., the immobility time) throughout the test was manually counted based on the recorded video. The rat was judged immobile when it persisted motionless and floating without struggling and when it moved merely to maintain its head above the water.
The Y-maze test assessed the spatial working memory after the last Pg-LPS injection following a described protocol [23]. Briefly, the rat was placed at the terminus of one arm of the maze apparatus (catalog no.YM-03R, Muromachi Kikai, Tokyo, Japan) and allowed to move freely across the maze for 8 min. The entry was regarded as complete when the tail of the rat was entirely within the arm. Alternation behavior was regarded as successive entries into all three arms of the maze on successive occasions. The percentage of spontaneous alternation behavior (SAB) was estimated by the formula mentioned below.
At 2 hr after the last administration of the Pg-LPS treatment, the rats in the immunohistochemistry group were sacrificed under deep intraperitoneal anesthesia with a mixture of three drugs: medetomidine (Domitor, Nippon Zenyaku Kogyo, Fukushima, Japan) at 0.15 mg/kg BW/rat, midazolam (Dormicum, Astellas Pharma, Tokyo, Japan) at 2 mg/kg BW/rat and butorphanol (Vetorphale, Meiji Seika Pharma, Tokyo, Japan) at 2.5 mg/kg BW/rat. Saline (Otsuka Pharmaceutical Factory, Tokushima, Japan) was then added up to adjust the mixture to a volume of 0.5 mL/100 g BW/rat. The rats were perfused transcardially with 500 mL of physiological saline and then 500 mL of 4% of paraformaldehyde (PFA) in phosphate buffer solution (Fujifilm, Wako, Osaka, Japan). After the perfusion, the brains were quickly removed and fixed with 4% of paraformaldehyde in phosphate buffer solution for 4 hr at room temperature (RT). The brains were submersed in 10% sucrose solution overnight at 4 °C and were subsequently immersed in 20% sucrose solution for 24 hr at 4 °C. The brains were sectioned at 40-µm thickness in the frontal plane by using a microtome (Microm HM 430, Thermo Scientific, Walldorf, Germany).
The free-floating brain sections were incubated in 1% H
The intensity of microglial and astrocytic immunoreactivity in DAB-stained
images was measured by a computer-assisted image analysis program (ImageJ 1.52a,
National Institutes of Health, Bethesda, MD, USA) described [24]. The images were captured
by using a BZ-X700 all-in-one microscope (Keyence, Osaka, Japan) with a
20
All the data are expressed as the mean
The behavioral tests were conducted to examine whether peripheral injection of
Pg-LPS can cause depressive-like behavior, such as behavioral despair
and impaired spatial working memory. In the FST, learned helplessness in the rats
was evaluated by measuring the immobility time, which was 108.73
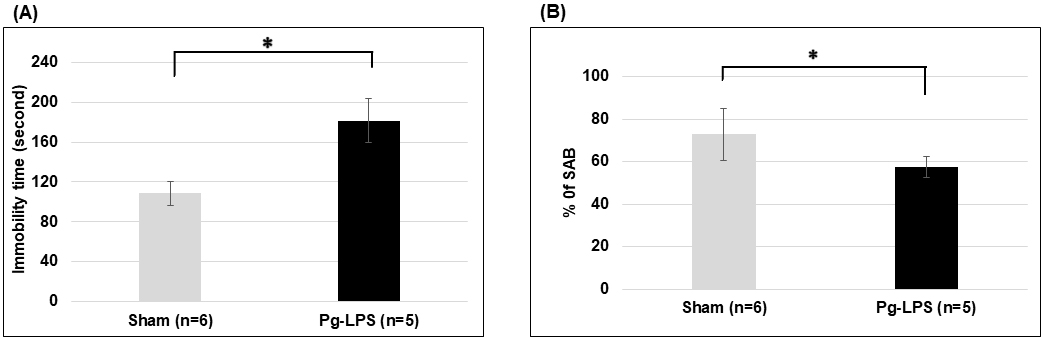
Behavioral tests of Pg-LPS-injected rats. (A) Effects
of Pg-LPS injection on immobility time in the forced swimming test. (B)
Effect of Pg-LPS injection on spontaneous alternation behavior (SAB) in
the Y-maze test. *p
Group | Mean |
p-value | |
Immobility time (sec) | Sham (n = 6) | 108.73 |
0.014 |
Pg-LPS (n = 5) | 181.54 | ||
% SAB | Sham (n = 6) | 72.83 |
0.028 |
Pg-LPS (n = 5) | 57.43 |
S.D., standard deviation; SAB, spontaneous alternation behavior; Pg-LPS, Porphyromonas gingivalis-lipopolysaccharide.
We evaluated the rat’s spatial working memory in the Y-maze test by calculating
the percentage of SAB. As shown in Fig. 2B. the percentage of SAB was 72.83
We investigated the immunoreactivity of microglial Iba-1 in the hippocampus
(CA1, CA3, and DG regions; Fig. 3A,B,D,E,G,H) and the PFC (PrL and IL regions;
Fig. 3J,K,M,N). Among these regions, the CA3 region showed significantly higher
immunoreactivity for Iba-1 in the Pg-LPS-injection group (18.05
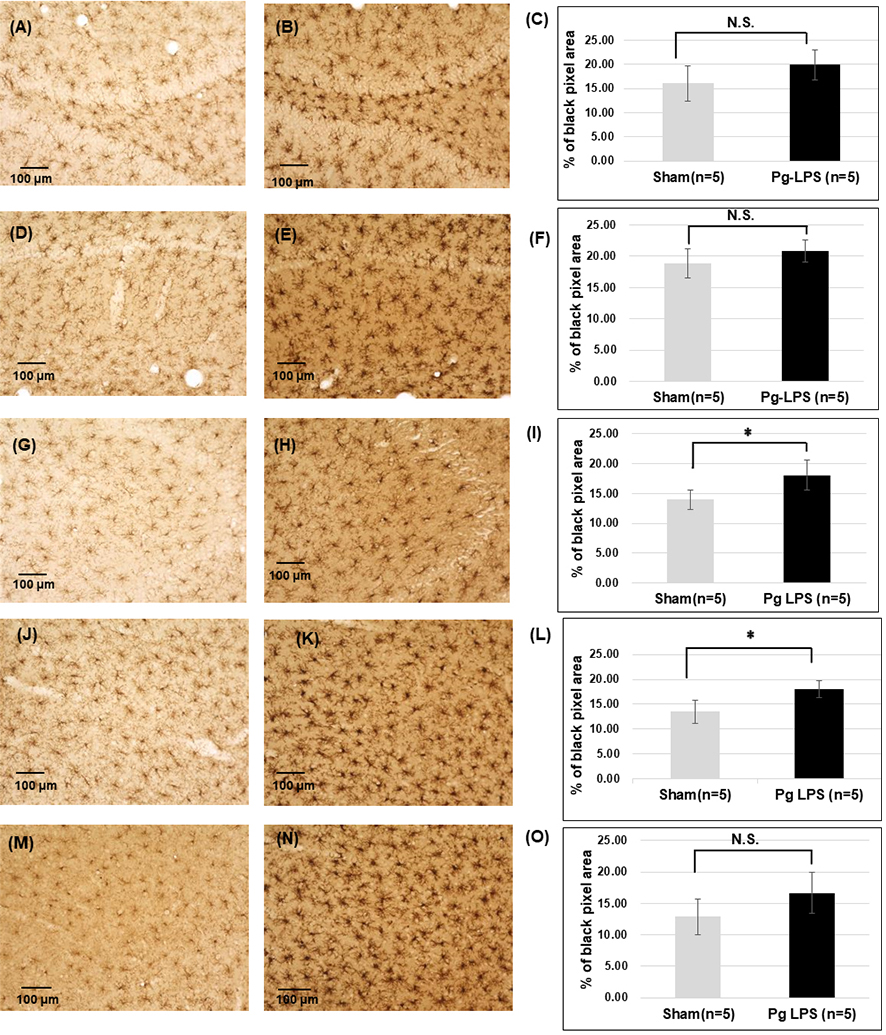
Immunoreactivity for Iba1 in the hippocampus and prefrontal
cortex. Representative images of 0.5% diaminobenzidine (DAB) staining in the
dentate gyrus (DG) of Sham (A) and Pg-LPS rats (B), the cornu ammonis
(CA)1 of Sham (D), and Pg-LPS rats (E), and CA3 of the Sham (G) and
Pg-LPS rats (H), prelimbic (PrL) area of Sham (J) and Pg-LPS
rats (K), and the infralimbic (IL) area of Sham (M), and Pg-LPS rats
(N). Scale bars, 100 µm; Quantification data of Iba1-immunoreactivity in
the DG (C), CA1 (F), CA3 (I), PrL (L), and IL (O). *p
Group | % of black pixel area (Mean |
p-value | |
DG | Sham (n = 5) | 16.09 |
N.S. |
Pg-LPS (n = 5) | 19.92 | ||
CA1 | Sham (n = 5) | 18.82 |
N.S. |
Pg-LPS (n = 5) | 20.79 | ||
CA3 | Sham (n = 5) | 13.95 |
0.014 |
Pg-LPS (n = 5) | 18.05 | ||
PrL | Sham (n = 5) | 13.49 |
0.008 |
Pg-LPS (n = 5) | 18.05 | ||
IL | Sham (n = 5) | 12.86 |
N.S. |
Pg-LPS (n = 5) | 16.65 |
S.D., standard deviation; DG, dentate gyrus; Pg-LPS, Porphyromonas gingivalis-lipopolysaccharide; CA, cornu ammonis; PrL, prelimbic; IL, infralimbic; N.S., not significant.
We also evaluated the astrocytic GFAP immunoreactivity in the hippocampus (CA1,
CA3 and DG regions; Fig. 4A,B,D,E,G,H) and the PFC (PrL and IL regions; Fig. 4J,K,M,N). The hippocampal CA1 regions showed significantly higher
immunoreactivity for GFAP in the Pg-LPS-injection group (27.98
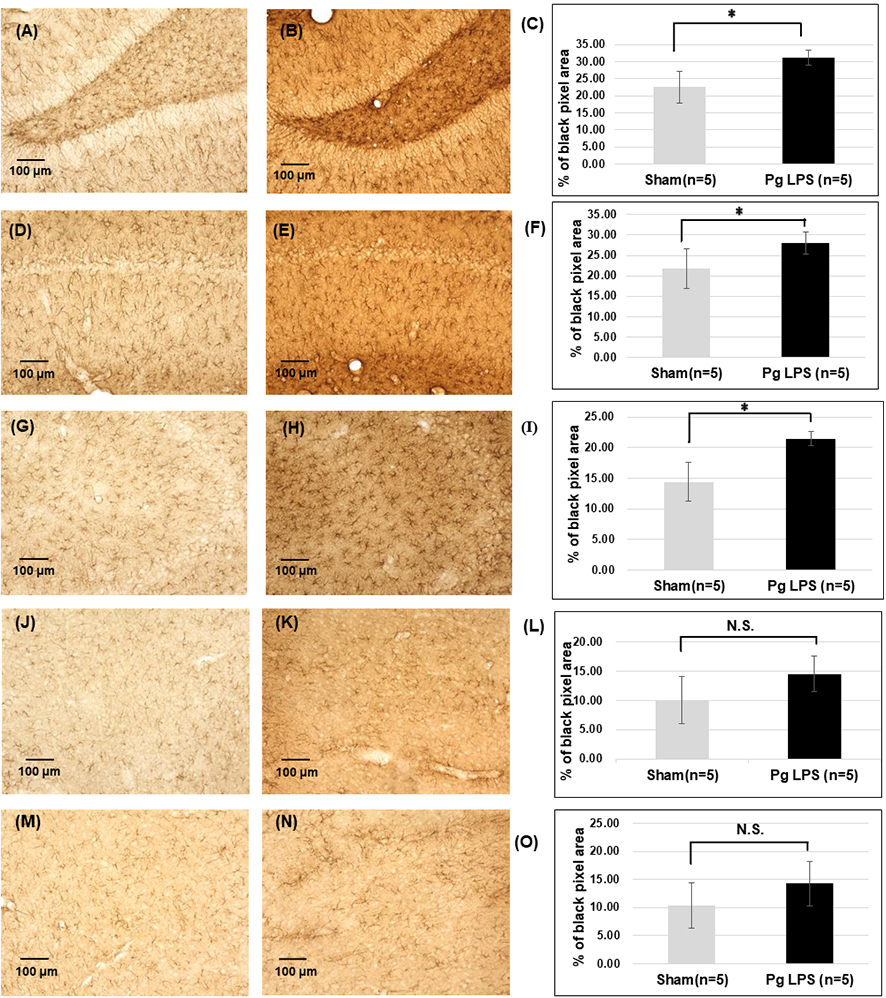
Immunoreactivity for GFAP in the hippocampus and the prefrontal
cortex. Representative images of DAB staining in the DG of Sham (A) and
Pg-LPS rats (B), CA1 of Sham (D) and Pg-LPS rats (E), CA3 of
Sham (G) and Pg-LPS rats (H), PrL of Sham (J) and Pg-LPS rats
(K), and IL of Sham (M), and Pg-LPS rats (N). Scale bars, 100 µm;
Quantification data of Iba1-immunoreactivity in the DG (C), CA1 (F), CA3 (I), PrL
(L), and IL (O). *p
Area | Group | % of black pixel area (Mean |
p-value |
DG | Sham (n = 5) | 22.59 |
0.006 |
Pg-LPS (n = 5) | 31.19 | ||
CA1 | Sham (n = 5) | 21.75 |
0.037 |
Pg-LPS (n = 5) | 27.98 | ||
CA3 | Sham (n = 5) | 14.41 |
0.002 |
Pg-LPS (n = 5) | 21.47 | ||
PrL | Sham (n = 5) | 10.06 |
N.S. |
Pg-LPS (n = 5) | 14.56 | ||
IL | Sham (n = 5) | 10.37 |
N.S. |
Pg-LPS (n = 5) | 14.28 |
S.D., standard deviation; DG, dentate gyrus; Pg-LPS, Porphyromonas gingivalis-lipopolysaccharide; N.S., not significant.
A major finding from the behavioral tests in the present study is that repeated i.p. injection of Pg-LPS elicited depressive-like behavior in SD rats. In the FST, the Pg-LPS-injected rats showed significantly longer immobility time compared to saline-injected rats. The immobility time represents learned helplessness and can be considered behavioral despair [22, 25]. This finding is consistent with an earlier investigation which demonstrated that mice treated intraperitoneally with Pg-LPS exhibited significantly prolonged immobility time in the FST [19]. The Pg-LPS-injected rats in the present study also showed a significantly decreased % of SAB in the Y-maze test. The decrease in SAB can be interpreted as impaired spatial working memory [26, 27]. Clinically, it has been observed that individuals with MD tend to have considerably impaired working memory [28]. Our present finding is also in line with a report that peripheral injection of Pg-LPS led to impaired spatial learning in mice [20]. The previous investigations of the effects of Pg-LPS on depressive-like behavior used mice [19, 20]; to the best of our knowledge, the present study is the first one in rats demonstrating that a peripheral administration of Pg-LPS induced behavioral despair and impaired spatial working memory.
A major finding of this study’s immunohistochemical analysis is that the systemic administration of Pg-LPS increased microglial Iba-1 immunoreactivity and astrocytic GFAP immunoreactivity in the MD-related brain regions, i.e., the hippocampus and PFC. Our finding of astrocytic activation in the hippocampal regions, as shown by raised GFAP immunoreactivity, is consistent with the above-cited studies using mice, which demonstrated that i.p. injection of Pg-LPS raised the number of GFAP-immunopositive cells in the hippocampus and PFC [19, 20]. We also observed a non-significant trend towards an increase in GFAP immunoreactivity in the PFC. Accordingly, the hippocampus may be more sensitive to the activation of astrocytes by Pg-LPS compared to the PFC.
Our finding of Pg-LPS-induced activation of microglia in the CA3 and PrL regions was verified by quantification of Iba-1 immunoreactivity, while an aforementioned study [20] described microglial activation based only on morphological inspection. The other study mentioned above [19] reported no significant change in the messenger RNA (mRNA) of the microglial markers Iba-1 and CX3CR1 in Pg-LPS-injected mice. Such discrepancy in the effects of Pg-LPS on the microglial state may stem from differences in the species and/or the concentrations of Pg-LPS applied.
It has been documented that E.coli-LPS containing lipid A with a conical shape induces the production of pro-inflammatory cytokines through Toll-like receptor (TLR) 4. On the other hand, Pg-LPS comprising more cylindrical lipid A, binds to TLR2 and elicits the cytokine production less efficiently than E.coli-LPS [29]. Moreover, Pg-LPS has been shown to induce a weaker neuroinflammatory response, compared with E.coli-LPS, in the rat circumventricular organs [30]. We, therefore, used higher doses of Pg-LPS in this study, relative to typical Pg-LPS doses (0.1–0.2 mg/kg) capable of causing depressive-like behavior in rats [31, 32].
It is elusive whether glial activation is exactly involved in the MD pathogenesis, as only a few studies have evaluated glial activation using postmortem brain samples from individuals with MD. Nevertheless, in vivo, studies demonstrated that mice with activated microglia and activated astrocytes, which were induced by peripheral injection of E. coli-LPS, exhibited depressive-like behavior [3, 33]. Our present finding verified that Pg-LPS also caused microglial activation, astrocytic activation, and depressive-like behavior in rats. Moreover, the results of one of our earlier investigations implied that both microglial activation and astrocytic activation in the hippocampus were related to depressive-like behavior in Gunn rats with congenital hyperbilirubinemia, another neuroinflammatory model associated with MD [34]. Taken together, our past and present findings support the hypothesis that glial activation may be closely correlated with presenting MD symptoms.
It has been suggested that dissociation between sickness behavior and depressive-like behavior attributes to time course in LPS-injected rodents [35]. Their sickness behavior, which can be considered neurovegetative symptoms (i.e., loss of appetite, insomnia, and fatigue), emerges first in response to LPS injection. Depressive-like behavior, which can be regarded as mood and cognitive symptoms, develops after the expression of sickness behavior. The limitation of this study is that changes in BW and sleeping time, which seem to reflect sickness behavior, were not evaluated.
Other limitations of the present study are as follows: First, we did not validate the immunohistochemistry findings via other methods, including gene expression profiling. Second, we did not perform behavioral tests on every rat. Third, Pg-LPS was injected into the peritoneal cavity, not into the gingiva. Accordingly, the experimental condition in this study does not exactly mimic periodontitis.
Our major finding is that a repeated systemic administration of Pg-LPS elicited depressive-like behavior, and both microglial and astrocytic activation could be biological evidence for a causal relationship between periodontitis-associated pathogens and MD.
All data generated or analyzed during this study are included in this published article.
RM: data curation, formal analysis, writing original draft, validation. SH: supervision, writing manuscript, data curation, validation, funding acquisition, project administration. IAA: data curation, validation. MAJ: data curation, validation. SJFJ: data curation, validation. KKK: validation. JF: data curation. KI: statistical analysis. MI: data curation. HT: supervision, validation. All authors contributed to editorial changes in the manuscript. All authors have read and agreed to the published version of the manuscript. All authors have participated sufficiently in the work and agreed to be accountable for all aspects of the work.
All experimental procedures were conducted and approved by the Shimane University Animal Ethics Committee, according to the guidelines of the National Health and Medical Research Council of Japan (Authorization No: IZ3-50).
Not applicable.
This study was supported by grants from the Japan Society for the Promotion of Science (JSPS) KAKENHI, Nos. 19K08018, 21K07537 and 22H02999.
The authors declare no conflict of interest.
Publisher’s Note: IMR Press stays neutral with regard to jurisdictional claims in published maps and institutional affiliations.