- Academic Editor
†These authors contributed equally.
Background: Delayed encephalopathy after acute carbon monoxide
poisoning (DEACMP) is a severe complication that can arise from acute carbon
monoxide poisoning (ACOP). This study aims to identify the independent risk
factors associated with DEACMP and to develop a nomogram to predict the
probability of developing DEACMP. Methods: The data of patients
diagnosed with ACOP between September 2015 and June 2021 were analyzed
retrospectively. The patients were divided into the two groups: the DEACMP group
and the non-DEACMP group. Univariate analysis and multivariate logistic
regression analysis were conducted to identify the independent risk factors for
DEACMP. Subsequently, a nomogram was constructed to predict the probability of
DEACMP. Results: The study included 122 patients, out of whom 30
(24.6%) developed DEACMP. The multivariate logistic regression analysis revealed
that acute high-signal lesions on diffusion-weighted imaging (DWI), duration of
carbon monoxide (CO) exposure, and Glasgow Coma Scale (GCS) score were independent risk factors for
DEACMP (Odds Ratio = 6.230, 1.323, 0.714, p
The global prevalence of acute carbon monoxide poisoning (ACOP) is 137 cases per million people, with a mortality rate of 4.6 cases per million people [1]. ACOP can result in dysfunction of multiple organs, particularly the cardiovascular and central nervous systems. Carbon monoxide (CO) poisoning can result in non-specific symptoms, with mild exposure leading to headache, myalgia, dizziness, or neuropsychological impairment, while severe exposure can cause more serious symptoms such as confusion, loss of consciousness, or even death [2, 3, 4]. Following ACOP, most affected patients recover from the acute stage of poisoning. However, in some cases, patients may experience a recurrence of neuropsychiatric symptoms after a latent period of normal or near-normal neurological function, which is commonly referred to as the “lucid interval” and typically lasts for 3–60 days. These recurring symptoms are collectively known as delayed encephalopathy after acute carbon monoxide poisoning (DEACMP) [5].
Although the mortality rate from ACOP is decreasing, the major concern following carbon monoxide poisoning is the risk of DEACMP in survivors [6, 7]. Care for patients with CO poisoning is not limited to preliminary measures. Accurate identification of patients with suspected neuropsychiatric injury, and the provision of drugs and rehabilitation therapy can help reduce the mortality of DEACMP [8]. Therefore, identifying risk factors and providing appropriate treatment are crucial in preventing DEACMP. Well-established independent risk factors for DEACMP include low initial Glasgow Coma Scale (GCS) score, CO exposure time, number of hyperbaric oxygen treatments (HBOT), and abnormal magnetic resonance imaging (MRI) findings during the acute phase [9, 10, 11, 12]. Additionally, laboratory indicators such as leukocyte count, myocardial enzyme levels, and troponin I levels have been found to predict DEACMP [11, 13, 14]. A nomogram, an intuitive graphical prediction model, can integrate several risk factors to provide accurate and individualized risk predictions, thereby enabling a more individualized prediction of prognosis and risk assessment for each patient [15]. However, currently, there is no accurate nomogram model available to assess the risk factors for DEACMP. Therefore, the aim of this study was to investigate whether acute high-signal lesions on diffusion-weighted imaging (DWI), routine blood test and clinical data characteristics could be identified as risk factors for DEACMP and to develop a nomogram for predicting the risk of DEACMP.
The case-control study included patients with acute carbon monoxide poisoning admitted to the emergency department (ED) of Yan’an People’s Hospital of Shaanxi Province from September 2015 to June 2021. This study was approved by the medical ethics committee of the hospital. The study was retrospective and the data extracted were anonymous, so the need for written informed consent was waived.
Our study included patients with ACOP who received treatment in the ED from September 2015 to June 2021.
The diagnostic criteria for ACOP were a positive qualitative result of carboxyhemoglobin (COHb) and an established history of CO exposure [7]. The inclusion criteria were as follows: (i) age 18–80, (ii) diagnosed as ACOP, (iii) suffered from ACOP for the first time, regardless of the cause of poisoning, (iv) did not participated in any other clinical studies within the last six months. Exclusion criteria were: (i) no MRI examination, (ii) persistent neurological symptoms at discharge, (iii) death in acute stage after poisoning, (iv) pregnant women, (v) transfer to hospital or discharge before assessment.
All ACOP patients who arrived in the ED were treated with 100% high-flow oxygen
therapy through a face mask or mechanical ventilator following endotracheal
intubation. Although emergency HBOT is not generally considered superior to
atmospheric oxygen therapy to prevent these sequelae, many poisoned patients,
even intentional poisoning, are treated with HBOT [16]. The main indications for
HBOT were loss of consciousness, neurological dysfunction, cognitive dysfunction
or severe acidosis [11]. Patients were treated with HBOT using a monoplace
hyperbaric chamber. The total HBOT time was 90 min per session, and the target
pressure was 2.5 standard atmospheres. Upon admission, all patients underwent
conventional magnetic resonance imaging (MRI) and diffusion-weighted imaging
(DWI) scans to assess the severity of brain injury. However, MRI is not performed
when patients or their agents disagree or when medical conditions prohibit MRI
scanning. The MRI scans were performed using a 1.5 Tesla scanner (GE Signa HDe
1.5T superconducting magnetic resonance, 14519YR3, GE Healthcare Japan Corporation, Tokyo, Japan). The MRI scans included DWI (repetition time (TR), 4450
ms; echo time (TE), 92.9 ms; field of view (FOV), 240 mm
Routinely, neurological and psychiatric evaluations were performed prior to discharge to screen for any potential neuropsychiatric disorders. Patients who did not exhibit neurological symptoms were informed of DEACMP symptoms prior to discharge and were required to visit the neurology department or ED for regular follow-ups after discharge. Furthermore, if any neurological symptoms arose before a scheduled visit, patients were advised to return to the hospital. Additionally, we conducted monthly telephone interviews with patients or their relatives to assess DEACMP. As reliable diagnostic criteria for DEACMP are currently lacking, we referred to previous studies [7, 12, 17]. The diagnostic criteria utilized in this study were the emergence of any new neurological symptoms or signs after recovery from neurocognitive symptoms resulting from acute CO poisoning. These symptoms included memory impairment, motor impairment, cognitive decline, dysarthria, Parkinson’s disease, incontinence, and others [17]. Finally, based on the presence or absence of DEACMP, patients were classified into two groups: the DEACMP group and the non-DEACMP group.
We retrospectively collected data on all patients from the electronic case management system, including sex, age, smoking habits, time intervals (coma time, CO exposure time, time interval from poisoning to MRI, time interval from poisoning to ED, time interval from poisoning to HBOT), treatment modality, number of HBOT before MRI scan, heart rate (HR), GCS score upon arrival, and MRI findings. Additionally, initial laboratory data were analyzed. For HR and laboratory indicators, we categorized the results into normal or abnormal (increased or decreased).
All statistical analyses were performed using IBM SPSS Statistics 18.0 (Version
18.0, IBM Corp., Armonk, NY, USA) and R software (version 3.6, Foundation for
Statistical Computing, Vienna, Austria). Continuous variables were expressed as
mean
A total of 181 patients with ACOP were initially identified. After applying inclusion and exclusion criteria, 122 patients were ultimately included in the study. Based on the presence or absence of DEACMP, 30 patients were assigned to the DEACMP group and 92 to the non-DEACMP group (Fig. 1).
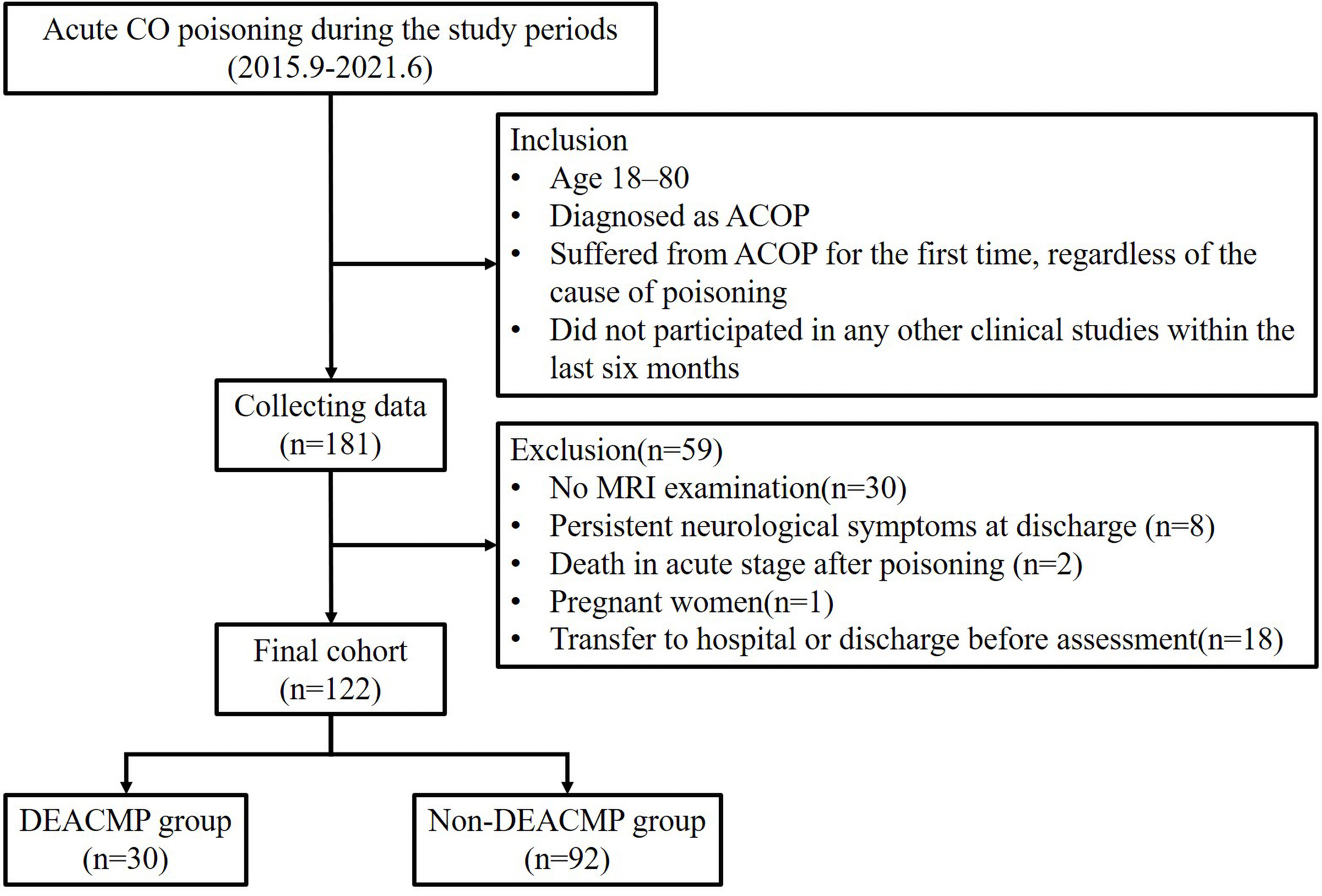
Flow diagram of the process of patient inclusion and exclusion in this study. ACOP, acute carbon monoxide poisoning; GCS, Glasgow coma scale; MRI, magnetic resonance imaging; DEACMP, delayed encephalopathy after carbon monoxide poisoning.
The baseline characteristics of the patients are presented in Table 1. Thirty
(24.6%) of the 122 patients developed DEACMP. There was no statistical
difference in sex, smoking habits, the time interval from poisoning to MRI or
time interval from poisoning to first aid between the two groups. The initial GCS
of the DEACMP group was significantly lower than that of the non-DEACMP group
(p
Variable | Total (n = 122) | DEACMP (n = 30) | Non-DEACMP (n = 92) | p-value | |
Gender, n (%) | 0.464 | ||||
Male | 46 (37.3%) | 13 (43.3%) | 33 (35.9%) | ||
Female | 76 (62.3%) | 17 (56.7%) | 59 (64.1) | ||
Age, years | 51.4 |
58.5 |
49.0 |
0.008* | |
Current smoking, n (%) | 28 (23.0%) | 8 (26.7%) | 20 (21.7%) | 0.577 | |
Coma duration, h | 1.0 (0.0–7.0) | 10.0 (6.0–20.0) | 0.1 (0.0–2.0) | ||
CO exposure time, h | 10.0 (5.0–11.0) | 13.0 (10.0–19.0) | 9.0 (5.0–10.0) | ||
Time interval from poisoning to ED, h | 2.0 (1.0–10.0) | 2.0 (1.0–3.0) | 2.0 (1.0–13.0) | 0.351 | |
Time interval from poisoning to MRI, h | 22.0 (14.0–96.0) | 25.0 (12.0–96.0) | 36.0 (24.0–96.0) | 0.227 | |
Time interval from poisoning to HBOT, h | 8.5 (4.0–24.0) | 6.0 (3.0–10.0) | 11.5 (4.0–26.0) | 0.010* | |
Treatment, n (%) | 0.149 | ||||
HBOT | 119 (97.5%) | 28 (93.3%) | 91 (98.9%) | ||
NBOT | 3 (2.5%) | 2 (6.7%) | 1 (1.1%) | ||
Number of HBOT before MRI scan | 2 (1–3) | 2 (1–2) | 2 (1–3) | 0.073 | |
HR ( |
17 (13.9%) | 4 (13.3%) | 13 (14.1%) | 0.913 | |
Initial GCS score | 12.5 (6.0–14.3) | 6.0 (4.8–7.0) | 14.0 (10.0–15.0) | ||
Abnormal Routine Blood Tests |
|||||
WBC ( |
62 (50.8%) | 24 (80.0%) | 38 (41.3%) | ||
NEUT% ( |
75 (61.5%) | 20 (66.7%) | 55 (59.8%) | 0.501 | |
LYMPH% ( |
77 (63.1%) | 21 (70.0%) | 56 (60.9%) | 0.368 | |
MONO% ( |
24 (19.7%) | 6 (20.0%) | 18 (19.6%) | 0.959 | |
RBC ( |
14 (11.5%) | 6 (20.0%) | 8 (8.7%) | 0.175 | |
HB ( |
45 (36.9%) | 16 (53.3%) | 29 (31.5%) | 0.032* | |
PLT( |
12 (9.8%) | 2 (6.7%) | 10 (10.9%) | 0.750 | |
High signal lesion on DWI, n (%) | 33 (27.0%) | 26 (86.7%) | 7 (7.6%) | ||
Hypertension, n (%) | 24 (19.7%) | 10 (33.3%) | 14 (15.2%) | 0.030* |
Count data are expressed as frequency (%) and measurement data are expressed as
means
p-value*, statistically significant.
a, all the continuous variables were transformed into categorical variables and
displayed as counts and percentages.
b, cut-off value men.
c, cut-off value women.
DEACMP, delayed encephalopathy after carbon monoxide poisoning; CO, carbon
monoxide; ED, emergency department; MRI, magnetic resonance imaging; HBOT,
hyperbaric oxygen therapy; NBOT, 100% normobaric oxygen therapy; HR, heart rate;
GCS, Glasgow coma scale; WBC, white blood cell; NEUT%, neutrophil percentage;
LYMPH%, lymphocyte percentage; MONO%, monocyte percentage; RBC, red blood cell;
HB, Hemoglobin; PLT, platelet; DWI, diffusion-weighted imaging.
Multivariate logistic regression analysis was performed with the statistically significant variables identified in the univariate analysis. The results revealed that acute high-signal lesions (OR 6.230, 95% CI 1.228–31.601, p = 0.027), CO exposure time (OR 1.323, 95% CI 1.034–1.693, p = 0.026), and Glasgow Coma Scale (GCS) score at admission (OR 0.714, 95% CI 0.556–0.916, p = 0.008) were independent risk factors for DEACMP (Table 2). In summary, prolonged CO exposure time increases the likelihood of DEACMP. Patients with high-signal nodules on DWI have an approximately 6-fold increased risk of developing DEACMP. A lower initial GCS score is associated with a worse mental state after poisoning, and an increased likelihood of subsequent delayed neurological symptoms. As a result, the equation of the model predicting DEACMP is:
Variable | OR | 95% CI (lower limit) | 95% CI (upper limit) | p-value |
CO exposure time | 1.323 | 1.034 | 1.693 | 0.026 |
High signal lesion on DWI | 6.230 | 1.228 | 31.601 | 0.027 |
GCS | 0.714 | 0.556 | 0.916 | 0.008 |
OR, odds ratio; CI, confidence interval.
Using CO exposure time, the presence of acute high-signal lesions on DWI, and GCS score at admission in multivariate analysis, a nomogram prediction model for DEACMP was developed (Fig. 2). The nomogram consists of 6 axes, with axes 2–4 representing each variable in the regression model. The performance of the nomogram was evaluated using the calibration curve and the ROC curve. The calibration curve demonstrated satisfactory consistency between the predicted probability of developing DEACMP by the nomogram and the actual probability of developing DEACMP (Fig. 3A). The ROC curve had an area under the curve (AUC) value of 0.959 (Fig. 3B), indicating good discrimination. DCA was used to evaluate the clinical efficacy of the predictive model, and the results showed that using the nomogram to predict DEACMP was more favorable than “no treatment” or “all treatment” strategies for threshold probabilities between 3% and 70% (Fig. 3C).
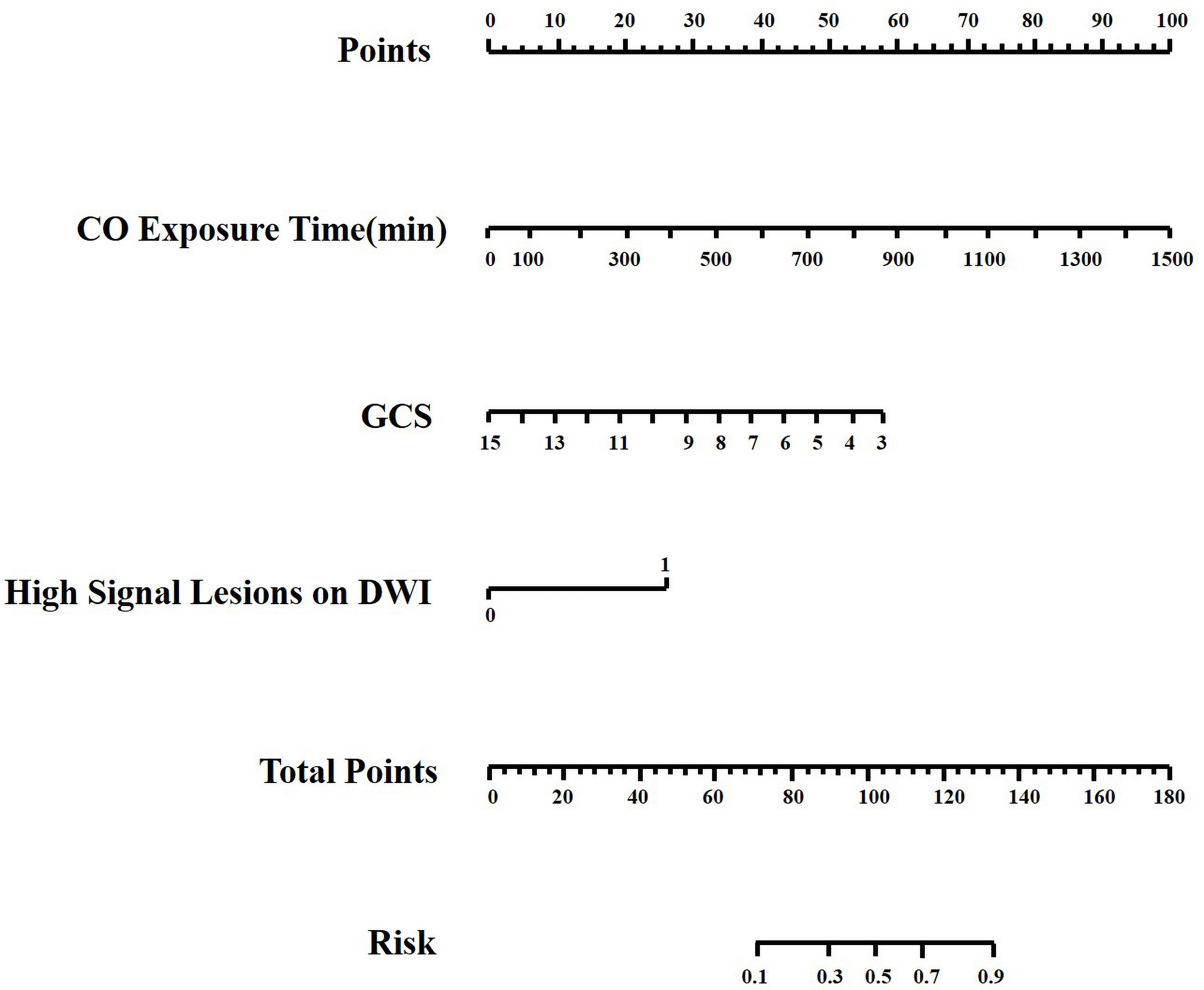
Nomogram for predicting the probability of delayed encephalopathy after carbon monoxide poisoning. Each variable value is assigned a point, and the sum of points is converted to a probability on the lowest scale. CO, carbon monoxide; GCS, Glasgow coma scale.
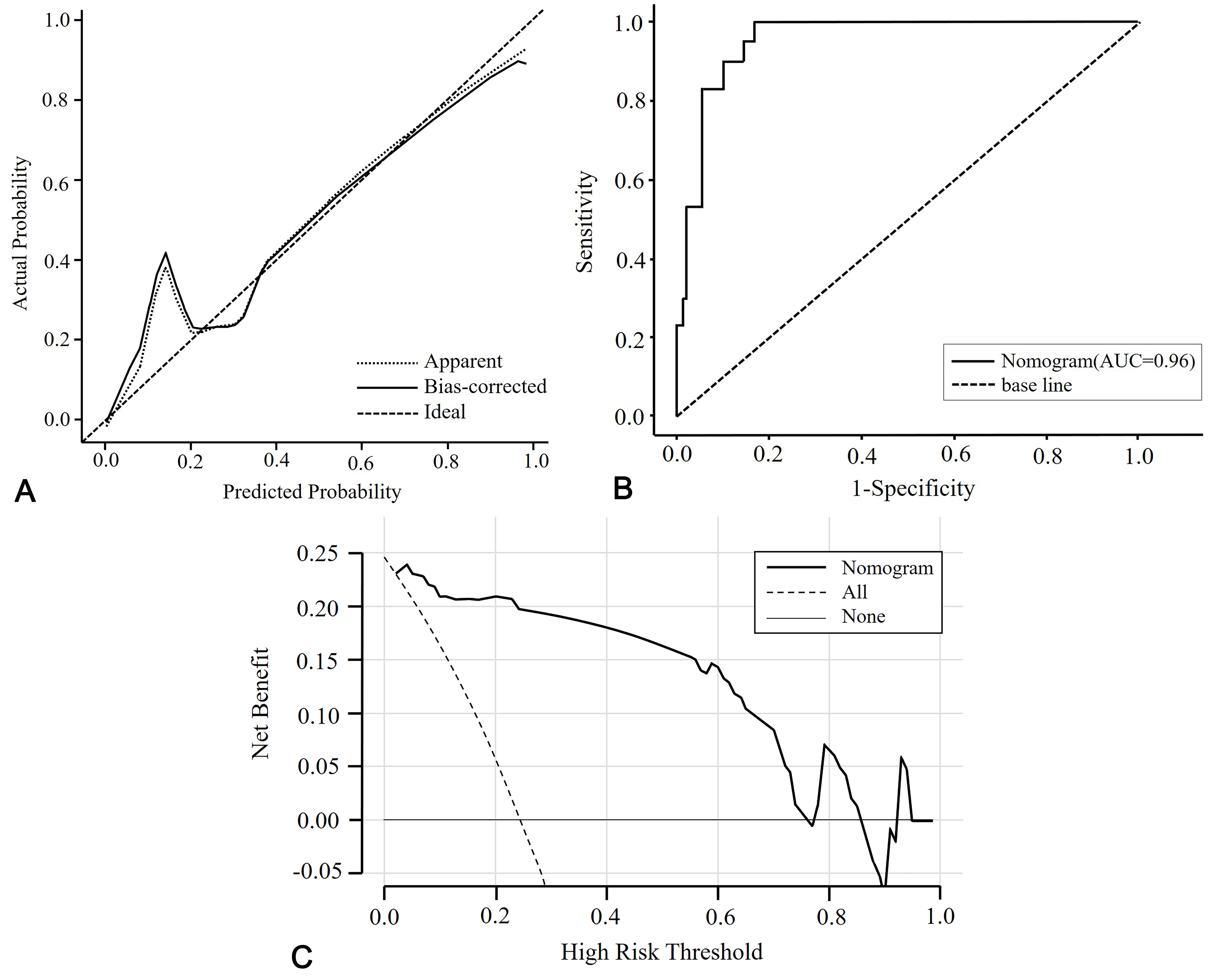
Evaluation of the nomogram. (A) Calibration curve of actual and predicted probabilities. The x-axis is the predicted probabilities measured by the final logistic regression model and the y-axis is the actual probabilities. (B) The ROC curve of the prediction model shows the predictive ability of DEACMP. The AUC value is 0.96. (C) Decision curve analysis for the nomogram. DEACMP, delayed encephalopathy after carbon monoxide poisoning; ROC, receiver operating characteristic; AUC, the area under the ROC curve.
In this retrospective study, we constructed and validated a nomogram to forecast the occurrence of DEACMP after ACOP. The prediction model formulated in this study demonstrates good accuracy in anticipating the possibility of future DEACMP development. Moreover, the DCA provided evidence for the potential clinical advantages of implementing this nomogram.
DEACMP typically presents as a delayed onset of neurocognitive symptoms following apparent recovery from acute CO poisoning, usually occurring between 2–60 days of sobriety. The complex mechanism of DEACMP occurrence has yet to be fully understood, leading to differences in reported DEACMP incidence among various studies [21, 22]. In our study, we observed that out of 122 patients with acute CO poisoning, 30 developed DEACMP, accounting for 24.6% of the total cases. Given the poor prognosis of DEACMP and its potential to significantly reduce patients’ quality of life, predicting the occurrence of DEACMP with high sensitivity is crucial to prevent the disease from worsening. Through the analysis of suspected risk factors for DEACMP, our study identified high signal lesions on DWI, GCS score, and CO exposure time as independent risk factors for DEACMP. These findings are consistent with those of previous studies [7, 17, 21, 23].
Several studies have suggested that high signal nodules on DWI can be used as an indirect marker for acute brain lesions and may predict the risk of DEACMP [7, 12, 24]. Jeon et al. [12] confirmed through multivariate analysis that altered mental state, prolonged CO exposure, and acute brain lesions were all independently associated with DEACMP. They also found that patients with high-signal nodules on DWI during the acute phase of poisoning had a 14-fold higher risk of DEACMP compared to patients without such nodules, regardless of their pattern, location, or region involved [12]. Another study indicated that patients with abnormal DWI results were six times more likely to develop DEACMP than those with normal results [7]. Accompanied by an increased prevalence of CO-induced hypoxic consequences during the acute phase, a more pronounced demyelination of cerebral white matter emerges, representing a pathological hallmark of DEACMP [25, 26]. Cognitive dysfunction constitutes the quintessential manifestation in patients afflicted with DEACMP [27]. Initial research accentuated the importance of not overlooking microvascular and diminutive macrovascular lesions within the purview of cognitive decline studies and interventions, as they represent the pivotal pathological element exacerbating cognitive deterioration [28, 29]. In this context, Parkinson and colleagues discovered that carbon monoxide poisoning can lead to brain injury, characterized by white matter hyperintensities in early-phase conventional MRI, with centrum semiovale hyperintensities being associated with poorer cognitive performance [30]. Therefore, based on our own study and previous research, it can be inferred that high-signal nodules on DWI have the potential to serve as a valuable screening tool for patients at a high risk of DEACMP, as they predict its occurrence more accurately by reflecting the extent of brain tissue injury. Moreover, due to the objectivity and high accuracy of DWI imaging and the simplicity of the imaging process, it is widely available in any hospital with MRI scanning equipment. In conclusion, as a powerful predictive tool for DEACMP, DWI has unique advantages. Further research is necessary to elucidate the complex mechanism underlying high-signal nodules and to establish a more solid relationship between high signal lesions on DWI and DEACMP.
A previous study has found that exposure to CO for a duration exceeding 6 hours increases the risk of DEACMP (OR, 1.28; 95% CI, 0.09–2.9) [11]. Additionally, another study conducted by Zhang et al. [31] concluded that patients exposed to CO for more than 4.8 hours had an increased possibility of developing DEACMP, as determined by ROC analysis. The findings of the current study are consistent with previous studies and suggest that prolonged CO exposure in patients increases the probability of DEACMP occurrence. As with other toxic gas poisonings, the extent of damage inflicted on the body due to CO poisoning mainly depends on the concentration of CO in the air and the duration of CO inhalation. A prospective observational study has suggested that shortening the duration of CO exposure may reduce the risk of DEACMP [7]. Thus, prolonged CO exposure is considered an independent risk factor for DEACMP.
In our study, the GCS score was found to be an independent risk factor for the development of DEACMP. The GCS score reflects the patient’s level of consciousness, with lower scores indicating poorer mental status and higher risk for future complications. Currently, various studies employ different GCS thresholds to evaluate the risk of DEACMP [9]. Furthermore, according to Zhang et al.’s study [31], both the GCS score at the exposure site and the GCS score in the emergency room were found to be predictors of DEACMP, with cut-off values of 7.5 and 10.5, respectively. The GCS score at the exposure site may be a more sensitive indicator of DEACMP, with a higher Youden index, compared to the GCS score in the emergency room. However, the majority of studies have only evaluated GCS scores after patients arrived at the hospital, with a cut-off value ranging between 9 and 13 [11, 32]. While one study did evaluate both on-site and emergency room GCS scores, the latter had a lower AUC (0.90 vs. 0.86) [31]. Several researchers have proposed that DEACMP is more likely to occur in patients with a GCS score of less than 9 [11, 33]. Thus, it is recommended that all first aid personnel become familiar with and conduct the GCS score assessment in the event of a suspected case.
However, some limitations should be noted. First of all, our study was a single-center study based on retrospective analysis, which may suffer from small sample size and selection bias. Secondly, there is no standardized diagnostic tool for DEACMP at present. More importantly, it is possible that we did not follow the patient long enough for the symptoms of DEACMP to occur during this period. Thirdly, the 6 mm slice thickness of the DWI may have caused us to miss some small nodules. In addition, our laboratory analysis of carboxyhemoglobin utilized a qualitative assessment method, and we did not have quantitative data on carboxyhemoglobin for our subjects. As a result, multicenter, large-scale randomized, double-blind trials may be required in the future to corroborate our findings.
In conclusion, this study has developed a nomogram that utilizes clinical indicators and high-signal lesions on DWI to predict the likelihood of DEACMP. This nomogram may serve as a dependable tool to identify patients at high risk for DEACMP and allow for personalized treatment to reduce its incidence.
The datasets generated for this study are available on request to the corresponding author.
SW and WH designed the research study. SW performed the research. ZZ and TS provided help on data collection. HL and HW analyzed the data. SW and WH wrote the manuscript. All authors contributed to editorial changes in the manuscript. All authors read and approved the final manuscript. All authors have participated sufficiently in the work and agreed to be accountable for all aspects of the work.
The study was conducted in accordance with the Declaration of Helsinki, and approved by the Ethics Committee of Yan’an People’s Hospital of Shaanxi Province (study no. 2021-01-021). The study was retrospective and the data were anonymous, so the need for written informed consent was waived.
We would like to thank the investigators for collecting the data and all patients for their cooperation.
This research received no external funding.
The authors declare no conflict of interest.
Publisher’s Note: IMR Press stays neutral with regard to jurisdictional claims in published maps and institutional affiliations.