- Academic Editor
†These authors contributed equally.
Background: Based on susceptibility-weighted imaging (SWI) visibility,
deep medullary vein (DMV) scores are related to white matter damage (WMD) in
patients with cerebral small vessel disease (CSVD). However, whether mechanisms
are associated with DMV changes is unclear. We examined extracellular fluid (ECF)
roles in white matter associations between DMV scores and white matter integrity
(WMI) in patients with CSVD. Methods: We examined magnetic resonance
imaging (MRI) and clinical data from 140 patients with CSVD. DMV scores (0–18)
were assigned on SWI according to DMV anatomic regions and signal
continuity/visibility. WMI and ECF volumes were evaluated using free water (FW)
and fractional anisotropy (FA) values by diffusion tensor imaging (DTI).
Results: DMV scores were independently associated with FA after
adjusting for vascular risk factors, age, white matter hyperintensity (WMH)
volume, and CSVD burden [
In older adults, cerebral small vessel disease (CSVD) is often implicated in dementia and cognitive impairment [1]. Despite its prevalence and negative impact on cognitive function, underlying CSVD mechanisms and effective treatments are limited. Previous studies have indicated that white matter damage (WMD) is a major contributor to cognitive impairment in CSVD [2, 3, 4], but the causes of such damage are not fully understood, multifaceted, and may include: low cerebral blood flow [5], blood-brain barrier leakage [6], neuroinflammation [7], and venous abnormalities [8]. These mechanisms have garnered significant research traction in recent years.
The deep medullary vein (DMV) complex is of particular interest and is the main venous blood drain in periventricular white matter and the corona radiata. DMVs are perpendicular to the ventricle, have limited variation, and are often used to examine deep venous changes in cerebral vascular disease. Studies have reported that magnetic resonance imaging (MRI)-derived DMV scores, based on DMV visibility on susceptibility-weighted imaging (SWI), are associated with the presence and burden of CSVD [9]. Subsequently, studies also find that DMV scores are related to cognitive impairment or dementia in individuals with CSVD [10]. According to previous studies, a higher DMV score is associated with venous lumen stenosis and venous hypertension [11], which may obstruct venous drainage in white matter and cause extracellular fluid (ECF) accumulation [12]. Increased ECF may facilitate harmful substance accumulation (e.g., plasma protein and metabolic waste) and, critically, cause WMD [13]. Therefore, we hypothesized that decreased DMV visibility was associated with WMD when mediated by increased ECF.
To evaluate ECF volumes, diffusion tensor imaging (DTI) is widely used in nervous-system-disease research [12, 14, 15]. According to the free water (FW) elimination 2-compartmental model [16], FW reflects ECF volume corrected for partial volume effects in cerebrospinal fluid, while fractional anisotropy (FA) indicates white matter integrity (WMI) after removing FW signals.
Therefore, CSVD is a significant health challenge for older adults; thus, deeper mechanistic insights are warranted. This study focused on the DMV complex in CSVD-associated WMD and investigated relationships between DMV scores, ECF volumes, and WMI using advanced multimodal magnetic resonance imaging (MRI) approaches.
The Institutional Review Board of our hospital approved the study, and patients
provided written informed consent. Between January 2022 and August 2022, imaging
and clinical data were prospectively collected from patients. Enrolled patients
had MRI scans to assess for ischemic attack, lacunar infarct (LI), or dizziness.
Inclusion criteria were: age
In patients, a multimodal MRI protocol included 3D T1 weighted imaging (T1WI), T2 Flair, T2 weighted imaging (T2WI), SWI,
and DTI sequences on a 1.5 Tesla scanner (MAGNETOM Aera, Syngo Platform VD13A,
Siemens Healthcare, Erlangen, Germany). Sequence parameters were: (1) SWI:
repetition time (TR) = 27 ms; echo time (TE) = 20 ms; flip angle = 10; slice
thickness = 2 mm; intersection gap = 0 mm; field of view (FOV) = 24
Two blinded and experienced neuroradiologists independently reviewed images, and disagreements were resolved by consensus.
From STRIVE guidelines [17], 4 CSVD MRI markers were identified: high-grade
perivascular spaces (HPVS), high-grade white matter hyperintensities (HWMH),
cerebral microbleeds (CMBs), and LIs. An LI finding indicated round or
ovoid-shaped lesions (3–15 mm in diameter), which appeared as hypointensities on
T1WI and hyperintensities on T2WI. HPVS were assessed as the maximum numbers of
perivascular space (PVS) in unilateral basal ganglia and were represented by
enlarged PVSs
Total CSVD magnetic resonance (MR) scores were calculated and ranged from 0–4 accordingly, based on an absence (0)/presence (1) of each of the 4 CSVD markers. For example, a CSVD MR score of 4 was assigned when all the markers were identified on MR images. WMH volume was calculated on T2 Flair images using the WMH mask segmented by FSL BIANCA.
SWI sequences were constructed (thickness = 10 mm) using minimum intensity projection, and DMV scores were assessed using constructed SWI images. To calculate scores, we selected 5 consecutive periventricular slices in patients which covered most DMVs, from the ventricle level above the basal ganglia to where ventricles disappeared.
Based on regional anatomy, the DMV complex was apportioned into 6 segments: frontal, parietal, and occipital regions bilaterally. In DMV scoring, we used a semi-quantitative approach [18] where each of the 6 segments were separately scored (0–3) based on observed continuity and visibility in SWI sequences (Fig. 1). Total DMV scores-individual scores summed from the 6 segments, ranged from 0–18. A DMV score = 0 indicated prominent DMV, while 18 suggested undetectable DMV.
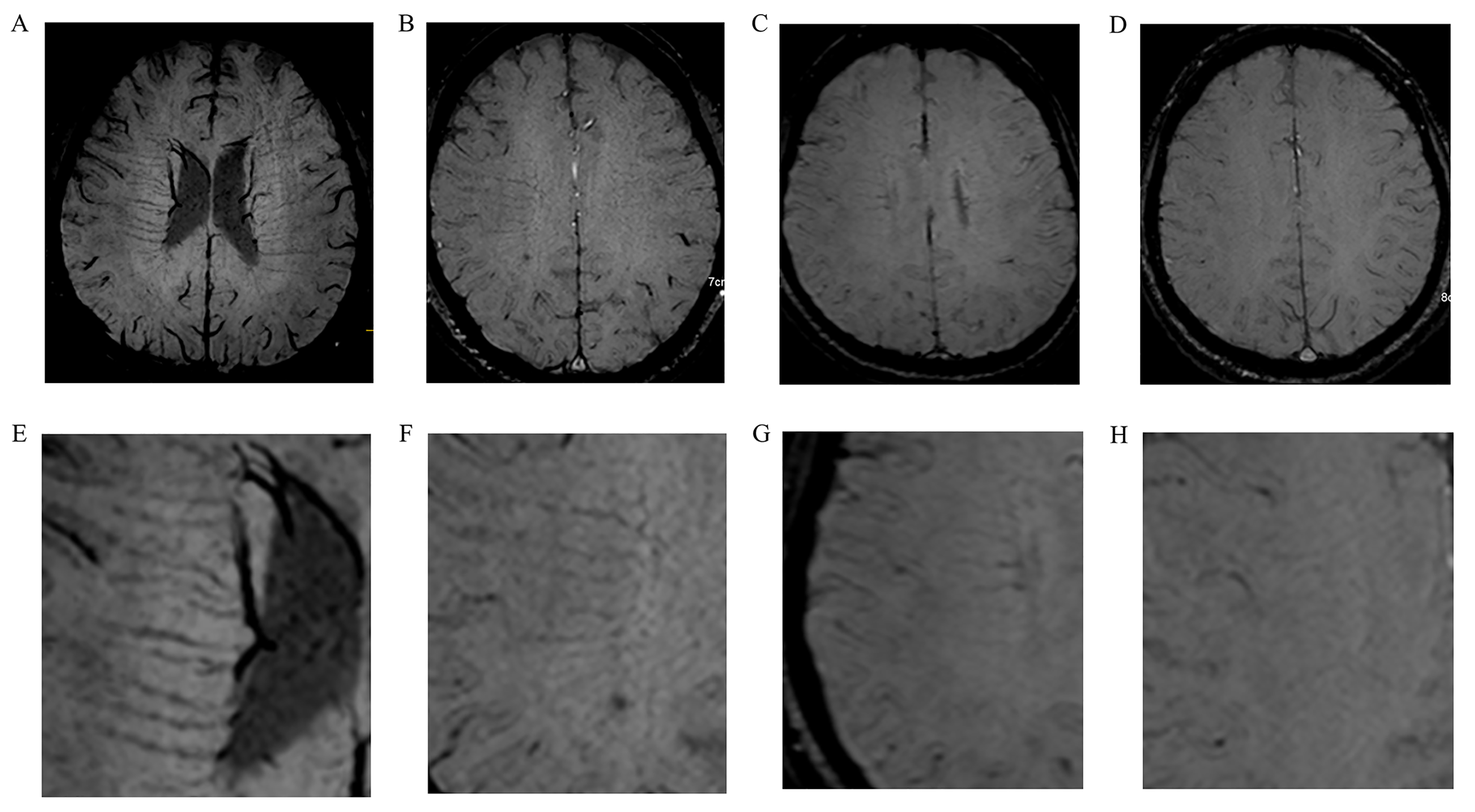
Typical images showing deep medullary vein (DMV) scoring. (A,E) DMV score = 0. The DMV signal is continuous and visible on susceptibility-weighted imaging (SWI). (B,F) DMV score = 1. The DMV signal is continuous with unequivocal visibility but has a non-homogeneous signal in at least 1 vein. (C,G) DMV score = 2. At least 1 vein is not continuous, is faintly visible, and contains spot-like hypointensities. (D,H) DMV score = 3. The DMV is invisible.
Gibbs artifact removal, denoising, echo planar imaging (EPI) distortion, and eddy current correction
pre-processing steps were conducted in Mrtrix3 software
(https://mrtrix.org/). Pre-processed DTI images were
analyzed for ECF and WMI in FW elimination 2-compartmental mode [19] (ECF and
tissue compartment) in DIPY software
(https://dipy.org/). FA and FW maps were generated, and
3D T1WI images co-registered with b = 0 s/mm
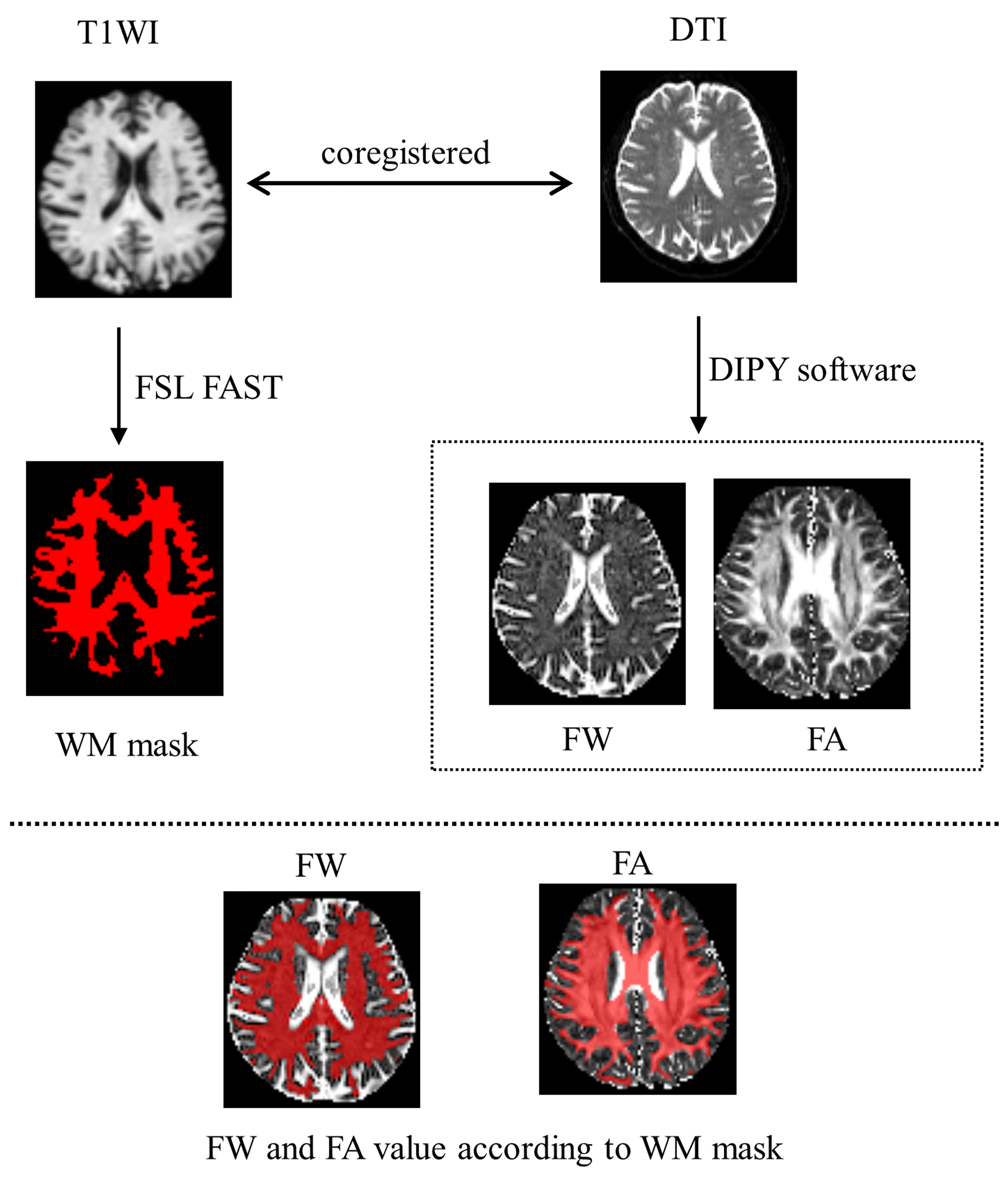
The processing pipeline showing image registration, white matter (WM) mask generation, and the calculation of free water (FW) and fractional anisotropy (FA) values. DTI, diffusion tensor imaging; T1WI, T1 weighted imaging; FSL FAST, FMRIB’s automated segmentation tool.
Categorical variables were represented as percentages and frequencies.
Continuous, normal distribution data were represented as the mean
We enrolled 140 patients (mean age = 60
Variables | n = 140 |
Male, n (%) | 66 (47.1) |
Age, years | 60 |
Hypertension, yes, n (%) | 76 (54.3) |
Diabetes mellitus, yes, n (%) | 25 (17.9) |
Current smoking, yes, n (%) | 33 (23.6) |
Hyperlipidemia, yes, n (%) | 36 (25.7) |
LI, yes, n (%) | 56 (40.0) |
HPVS, yes, n (%) | 20 (14.3) |
HWMH, yes, n (%) | 32 (22.9) |
CMB, yes, n (%) | 53 (37.9) |
WMH volume, mL | 12.29 |
DMV score, median (IQR) | 3 (0, 7) |
FW | 0.25 |
FA | 0.56 |
LI, lacunar infarct; HPVS, high-grade perivascular spaces; HWMH, high-grade white matter hyperintensities; WMH, white matter hyperintensities; CMB, cerebral microbleed; DMV, deep medullary veins; FW, free water; FA, fractional anisotropy; IQR, interquartile range.
As indicated (Fig. 3), Spearman’s correlation coefficients identified a
significant correlation between DMV scores and FA values in white matter (r =
–0.251, p
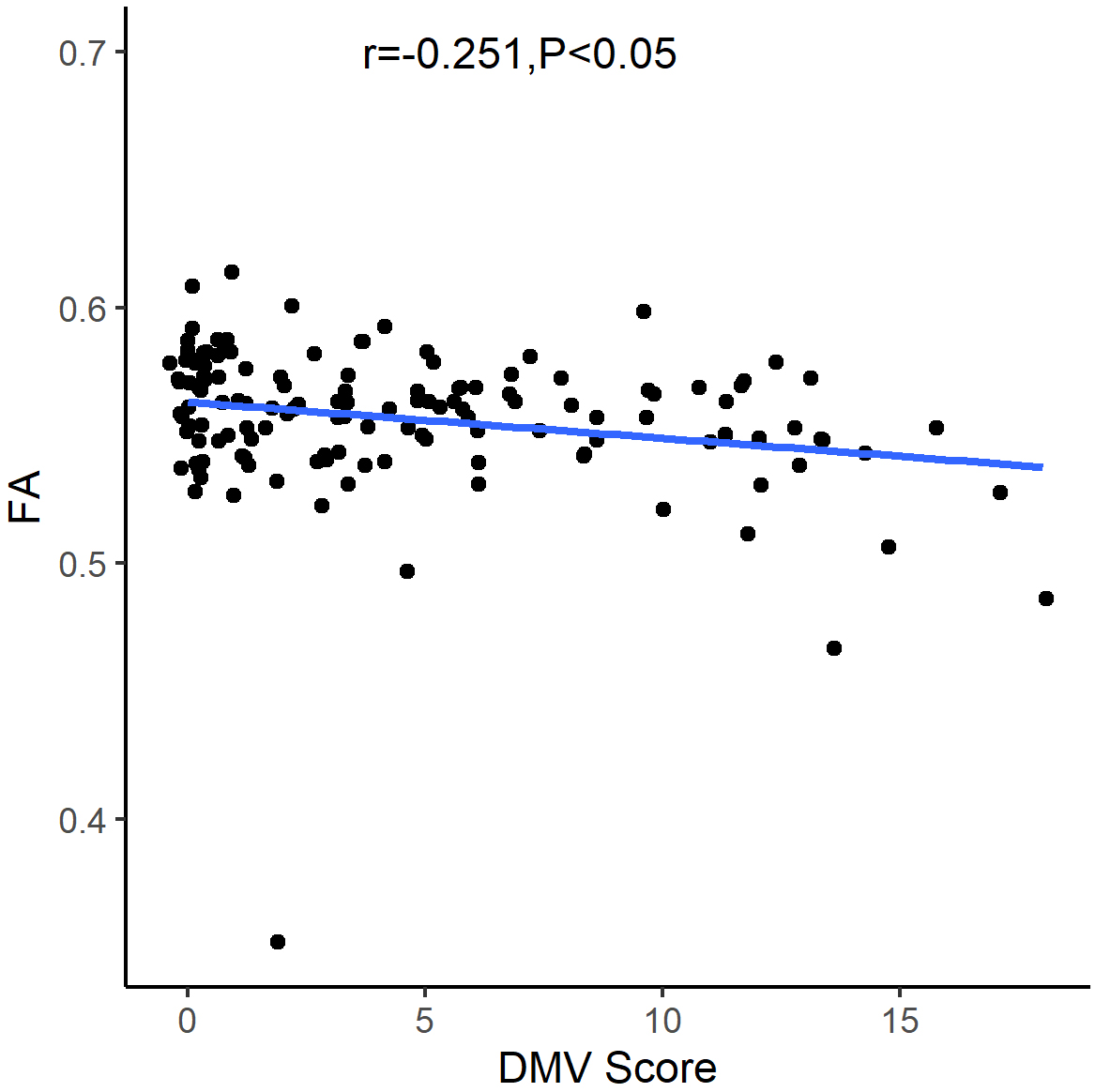
Graph showing the relationship between deep medullary vein (DMV) scores and fractional anisotropy (FA) values.
Variables | p value | |||
Lowest | Highest | |||
Age | –0.046 | –0.226 | 0.133 | 0.610 |
Hypertension | 0.031 | –0.116 | 0.179 | 0.677 |
Diabetes mellitus | –0.104 | –0.245 | 0.036 | 0.143 |
Current smoking | 0.110 | –0.026 | 0.245 | 0.111 |
Hyperlipidemia | 0.045 | –0.092 | 0.183 | 0.515 |
Total CSVD MR score | –0.020 | –0.200 | 0.159 | 0.825 |
WMH volume | –0.085 | –0.257 | 0.087 | 0.331 |
DMV score | –0.219 | –0.375 | –0.063 | 0.006 |
CSVD, cerebral small vessel disease; CI, confidence interval; MR, magnetic resonance.
As indicated (Fig. 4A), Spearman’s correlation coefficient analyses identified
significant correlations between DMV scores and FW values in white matter (r =
0.425, p
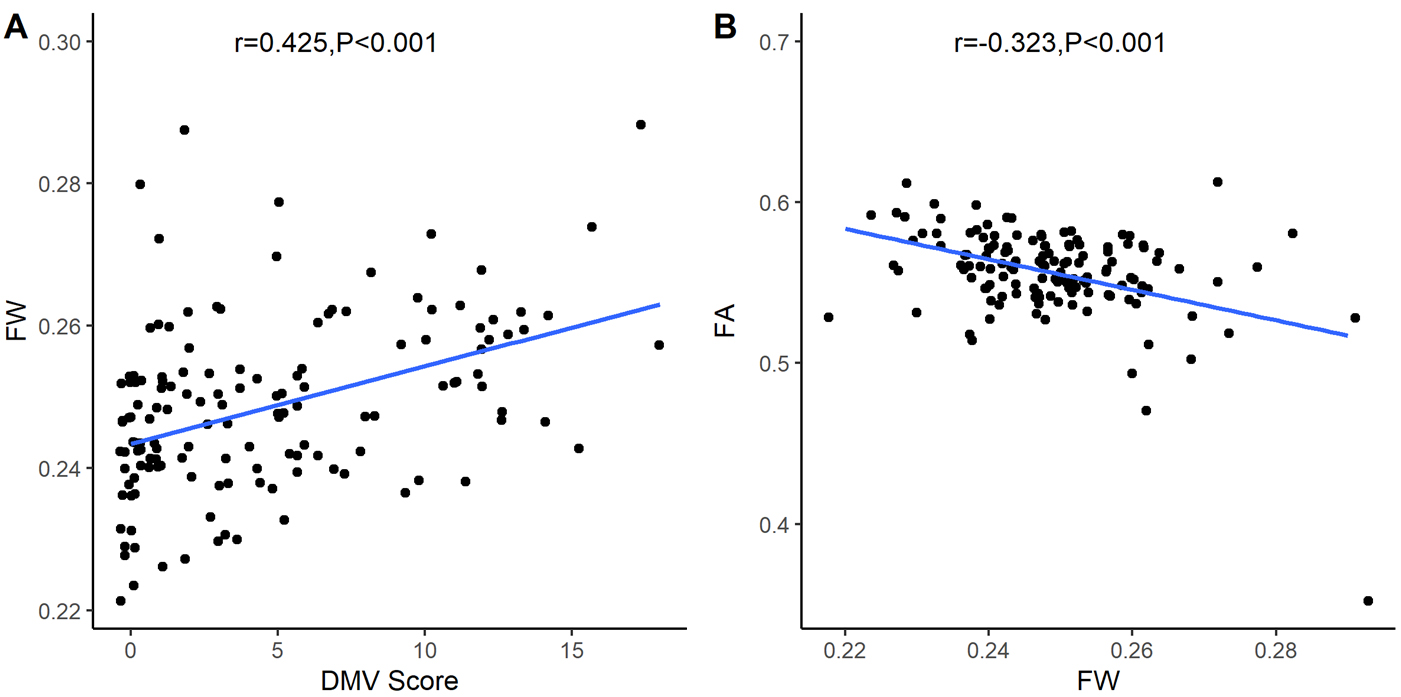
Graphs showing relationships between deep medullary vein (DMV)
scores, fractional anisotropy (FA), and free water (FW) values. A: There is a significant correlation between
DMV scores and FW values in white matter (r = 0.425, p
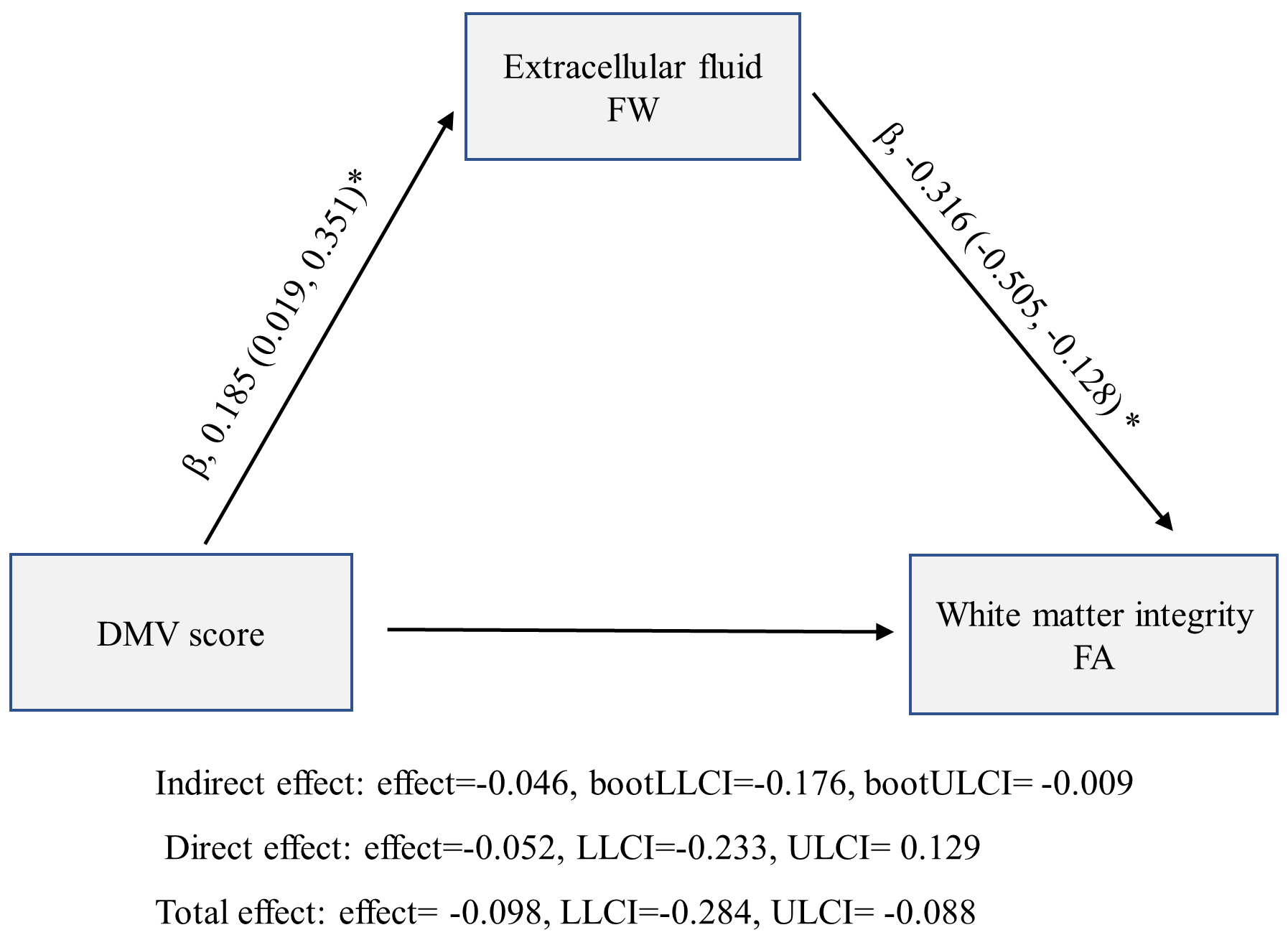
Mediation model analyses exploring relationships between deep
medullary vein (DMV) scores, fractional anisotropy (FA), and free water (FW)
values (*p
As indicated (Fig. 4B), Spearman’s correlation coefficient analyses identified
significant correlations between FW and FA values in white matter (r = –0.323,
p
Mediation analyses were controlled by age, vascular risk factors, WMH volume, and CSVD burden and identified significant indirect effects of DMV scores toward FA in the white matter when mediated by FW in white matter (Fig. 5).
DMV scores were independently associated with FA values in white matter and mediated by FW values in patients with CSVD. Also, mediation effects were independent of vascular risk factors, age, WMH volume, and CSVD burden. Thus, relationships existed between DMV damage, increased ECF, and WMI loss in patients with CSVD.
With rapid improvements in MRI technology, SWI is widely used to investigate cerebral vascular and neurodegenerative diseases [20, 21]. Many studies have reported that MRI-based DMV scores may be correlated with CSVD presence and severity [9, 18, 22]. Higher DMV scores are putatively correlated with a higher CSVD probability and severity. Previous autopsy and animal studies indicated that DMV damage may be caused by collagen type I and III deposition on vessel walls, leading to a stenotic or occluded lumen [11, 23, 24]. Higher DMV scores may reflect this DMV damage, and DMV abnormalities may be implicated in CSVD development.
DMV scores correlated with cognitive impairment in patients with CSVD [10], while associations between microstructure changes and WMI loss and cognitive impairment were reported in these patients [2]. However, the relationship between DMV damage and WMI loss and the precise influence of DMV scores on WMD remains unclear. Here, we identified links between DMV damage, increased ECF, and WMI loss in patients with CSVD. When a DMV abnormality was present, increased ECF initially occurred, followed by white matter demyelination and axonal damage.
Based on DMV structure and function, we suggest that DMV-associated white matter abnormalities occur when the DMV lumen narrows and venous pressure increases, causing obstructed venous drainage and increased vascular permeability [11], with increased PVS and ECF leakage volumes. It was previously believed that the index of diffusion tensor image along the PVS near the DMV was related to glymphatic system function [25]. Thus, a DMV abnormality may have caused glymphatic system dysfunction and increased ECF volume. However, venous hypertension may also have decreased cerebral blood flow and vascular edema, thereby increasing ECF. Either way, increased ECF volume may have facilitated harmful substance (e.g., plasma proteins and metabolic waste) accumulation, which elicited neuroinflammation, and further increased the ECF volume, thus ensuring a vicious circle. These substances can potentially damage white matter microstructures (e.g., myelin and axons), leading to WMI loss [13, 26]. In considering WMI loss and cognitive impairment evidence, identifying DMV abnormality and cognitive impairment steps may uncover new CSVD venous-related prevention and control mechanisms.
CSVD is related to aging and associated with one or more vascular risk factors [27]. WMH volume and CSVD burden were often correlated with more severe WMD [28]. Therefore, in mediation analyses, we adjusted for age, vascular risk factors, WMH volume, and CSVD burden. This approach enhanced the practicality of our findings and suggested that cerebral venous abnormalities initially occurred with increased ECF volume, followed by WMD. Furtherly, this observation was consistent with other research [8], which confirmed links between DMV scores and FW and FA values in patients with CSVD. Although research has shown a correlation between WMH volume, CSVD burden, age, and cognitive decline [3, 29], this study reveals that they are not independently associated with FA or WMI. The possible explanation is that the underlying mechanism driving cognitive decline or WMI involves changes in the brain’s microenvironment and microstructure, contributing to the development of WMH volume and CSVD burden.
Our study was limited. It was conducted at a single center and had a small sample. Secondly, no follow-up was conducted to examine relationships between DMV scores, FW and FA values, and cognitive impairment. Thirdly, low cerebral blood flow and inflammatory edema may also increase FW [28, 30]; however, we did not include these factors or adjust for them in our models, thus introducing potential bias. Fourthly, although the DMV scores system has now been used in the serial study, the software automatically detecting and measuring DMV scores may be better to quantitatively assess DMV changes. We mainly focused on extracellular FW, while intracellular water content was not analyzed. Finally, capillary blood perfusion may have affected extracellular FW values. Thus a 3-compartment model may improve FW estimations. To address the limitations mentioned above, more multi-center investigations with more patients are required.
DMV scores were independently associated with WMI and mediated by ECF volume in white matter in patients with CSVD. This observation may underpin a venous element of WMD pathogenesis in CSVD.
SWI, susceptibility-weighted imaging; DMV, deep medullary vein; CSVD, cerebral small vessel disease; DTI, diffusion tensor imaging; FW, free water; FA, fractional anisotropy; HWMH, high-grade white matter hyperintensities; CMB, cerebral microbleeds; HPVS, high-grade perivascular spaces; LI, lacunar infarcts; WMD, white matter damage; WMI, white matter integrity.
Study datasets are available upon reasonable request from the corresponding author.
HW, XL and ZX designed the research. HW, HZ, ZC, YW and HL performed the research. HL and HZ assisted with the research. HW and ZX analyzed data. HW, XL and ZX wrote the manuscript. All authors contributed to editorial changes in the manuscript. All authors read and approved the final manuscript. All authors have participated sufficiently in the work and agreed to be accountable for all aspects of the work.
The study was conducted according to the Declaration of Helsinki and approved by the Institutional Review Board of Tongde Hospital of Zhejiang Province (2022-042-JY). Written informed consent was provided prior to participation.
We thank our colleagues in the department of radiology for their support and assistance during data collection.
This research was funded by the Medical and Health Science and Technology Program of Zhejiang Provincial Health Commission of China, grant number 2022KY707.
The authors declare no conflict of interest.
Publisher’s Note: IMR Press stays neutral with regard to jurisdictional claims in published maps and institutional affiliations.