- Academic Editor
†These authors contributed equally.
Background: Bone cancer pain (BCP) is a common primary or metastatic bone cancer complication. Netrin-1 plays an essential role in neurite elongation and pain sensitization. This study aimed to determine the role of netrin-1 from the metastatic bone microenvironment in BCP development and identify the associated signaling pathway for the strategy of BCP management. Methods: The rat BCP model was established by intratibial implantation of Walker 256 cells. Von Frey filaments measured the mechanical pain threshold. Movement-induced pain was assessed using limb use scores. Expressions of associated molecules in the affected tibias or dorsal root ganglia (DRG) were measured by immunofluorescence, immunohistochemistry, real-time quantitative polymerase chain reaction, or western blotting. Transduction of deleted in colorectal cancer (DCC) signaling was inhibited by intrathecal injection of DCC-siRNA. Results: In BCP rats, the presence of calcitonin gene-related peptide (CGRP)-positive nerve fibers increased in the metastatic bone lesions. The metastatic site showed enrichment of well-differentiated osteoclasts and expressions of netrin-1 and its attractive receptor DCC. Upregulation of DCC and increased phosphorylation levels of focal adhesion kinase (FAK) and Rac family small GTPase 1/Cell division cycle 42 (Rac1/Cdc42) were found in the DRG. Intrathecal administration of DCC-siRNA led to a significant reduction in FAK and Rac1/Cdc42 phosphorylation levels in the DRG, decreased nociceptive nerve innervation, and improved pain behaviors. Conclusions: Netrin-1 may contribute to the activation of the BCP by inducing nociceptive nerve innervation and improving pain behaviors.
About 60–84% of patients with late-stage cancer experience different degrees of pain [1, 2]. Currently, bone cancer pain (BCP) is primarily treated using a 3-step analgesic ladder, as the World Health Organization outlined in 1986. Additional therapies, such as radiation therapy, nerve lesions, osteoprotegerin, and diphosphonate, may also be utilized [1, 3, 4]. However, due to the complex and interactive nature of BCP mechanisms, the available treatment options are limited [1, 5]. Therefore, it is crucial to gain a deeper understanding of the pathogenesis of BCP and explore alternative therapeutic targets for pain management.
BCP is believed to be caused by tumor-induced injury to sensory nerve fibers and abnormal sprouting of peripheral nociceptive nerve fibers in metastatic bone lesions [6, 7]. Recently, nociceptive neurons in cancer-affected bones have displayed increased elongation and innervation. The majority of these sprouting nerve fibers are composed of calcitonin gene-related peptide (CGRP)-fiber and are connected to dorsal root ganglia (DRG). These fibers release CGRP from their central terminals in the dorsal horn [8]. Accumulative evidence has documented that bone tissue is predominantly innervated by the peptide-rich CGRP + nerve fibers, which have been shown to participate in pain sensation [9, 10, 11, 12, 13]. The mechanisms leading to increased nociceptive nerve innervation at the site of bone metastases are poorly understood. In addition, the factors in the bone metastatic microenvironment that are thought to be involved in this pathological process require further investigation.
Netrin-1 is a neuronal guidance molecule that participates in developing the central nervous system and the regeneration of spinal cord and peripheral nerves [14, 15]. It possesses multiple physiological functions such as axonal guidance [16, 17], cell migration [18], morphogenesis [19], angiogenesis [20], and neuroinflammation [21]. Emerging studies have revealed the crucial role of netrin-1 in pain sensation by inducing the sprouting of nociceptive nerve fibers. Specifically, Wu et al. [14] demonstrated that netrin-1 increases deleted in colorectal cancer (DCC) expression in the spinal dorsal horn in resiniferatoxin-induced postherpetic neuralgia (PHN)-like rat model, contributing to mechanical hypersensitivity and sprouting of myelinated sensory fibers. Zhu et al. [22] proved that netrin-1 promotes sensory innervation and osteoarthritis pain in an osteoarthritis mouse model. Moreover, Ni et al. [23] found that osteoclast-secreted netrin-1 initiates the porosity of endplates and sensory innervation in a lumbar spine instability (LSI) mouse model and contributes to spinal pain.
Secreted by multiple types of cells, such as neurons [24, 25], macrophages [26], fibroblasts [27], and osteoclasts [22], netrin-1 accomplishes its physiological bi-functionality in nerve regeneration by binding to its canonical attractive receptors deleted in colorectal cancer (DCC) and neogenin or the repulsive DCC/uncoordinated (UNC5) A–D receptor complexes [17, 28]. Subsequently, the downstream signal of netrin-1/DCC signaling is responsible for neurite elongation, the phosphorylation of focal adhesion kinase (FAK), and following phosphorylation of Rac family small GTPase 1/Cell division cycle 42 (Rac1/Cdc42) play critical roles in axon navigation [29, 30].
As discussed previously, netrin-1 plays a potential role in developing and maintaining pain. In this study, we observed an elevated presence of CGRP + nerve innervation within the microenvironment of metastatic bone in a rat model of bone cancer pain (BCP), accompanied by a substantial differentiation of osteoclasts and expression of netrin-1. Furthermore, downstream netrin-1 signals, which promote neurite elongation through interaction with its attractive receptor DCC, were activated at the metastatic site and in the ipsilateral DRG of BCP rats. Subsequent knockdown of DCC via intrathecal injection of DCC-siRNA impeded the transduction of downstream signals associated with the netrin-1/DCC signaling pathway. It significantly alleviated the pain-related behaviors in BCP rats. These findings suggest that activating the netrin-1/DCC signaling pathway in cancer may subsequently contribute to an increased presence of nociceptive nerve fibers within the affected bone tissues, thereby contributing to the development of BCP.
Adult female Sprague-Dawley rats (6–8 weeks of age, Shanghai JieSiJie Laboratory Animals, Shanghai, China) were housed under standard laboratory conditions (light/dark cycle of 12/12 h, temperature 22–26 °C, humidity 55–60%) with water and food pellets available ad libitum. All experiments followed protocols approved by the Animal Care and Use Committee of Shanghai Chest Hospital, Shanghai Jiao Tong University, School of Medicine [permission no. KS (Y)20186] and the guidelines for investigations of experimental pain in animals published by the International Association for the Study of Pain [31].
Walker256 breast cancer cells (LLC-WRC 256, ATCC, Richmond, VA, USA), certified
by short tandem repeat (STR) analysis and tested for mycoplasma contamination,
were cultured in a 6 mL medium containing M199 (M4530, Sigma Aldrich, Steinheim
am Albuch, Germany), penicillin-streptomycin solution (100
The BCP model was developed by a single, well-trained male researcher utilizing
a rat breast cancer cell line (Walker256 cells), as detailed earlier [32].
Briefly, rats were randomly grouped and received an injection of
10 µL of Walker256 cells
(5
One male observer was blinded to the experimental design for the duration of the entire study and administered all behavioral tests.
A standard von Frey test was used to assess mechanical pain. Rats were placed in
Individual translucent brown plastic cages (20
Movement-induced pain was estimated using limb use scores [11, 33]. The rats
were permitted to move spontaneously on a smooth plastic table (50
cm
The ipsilateral tibia of BCP rats was fixed in 4% paraformaldehyde (P1110, Solarbio, Beijing, China) for 48 h, then decalcified in 10% ethylenediamine tetraacetic acid (EDTA; G1105, Servicebio, Wuhan, China) for 3–4 weeks, and 6-µm sections were cut using a rotating microtome. Paraffin-embedded sections were deparaffinized in xylene and rehydrated. Hematoxylin–eosin (H&E; GP1031, Servicebio, Wuhan, China) and Tartrate-resistant acid phosphatase (TRAP; G1050, Servicebio, Wuhan, China) staining were conducted according to the manufacturer’s protocol.
The tibias of sham-treated and BCP rats were collected and scanned with a VivaCT 80 scanner with a 55-kVp source (Scanco, Southeastern, PA, USA). The area of interest was the proximal 1/3 of the tibias. Images and parameters were acquired for 3D reconstruction, such as bone volume/total volume (BV/TV) and connectivity density (Conn.D.), using Scanco Medical software (Scanco mCT 40; SCANCO Medical AG, Bruttisellen, Switzerland).
The paraffin-embedded sections were deparaffinized in xylene (G1128, Servicebio,
Wuhan, China) and rehydrated. Antigen retrieval was performed with protease K
(G1234, Servicebio, Wuhan, China) at 37 °C for 15 min. Then the sections
were permeabilized in 0.3% Triton X-100 solution (P0096, Beyotime, Shanghai,
China) for 10 min, blocked with blocking solution (5% BSA (GC305006, Servicebio,
Wuhan, China) and 10% goat serum (G1208, Servicebio, Wuhan, China)) for 1 h and
finally incubated with primary antibodies against CGRP (1:100, sc-57053; Santa
Cruz Biotechnology, Santa Cruz, CA, USA), netrin-1 (1:100, PA5-95725, Invitrogen,
Carlsbad, CA, USA), DCC (1:1000, ab273570; Abcam, Cambridge, UK) and secondary
antibody (1:500) (catalog nos. #4412 and #8890, Cell Signaling Technology,
Boston, MA, USA) in the dark. Then an antifade mounting medium containing
4
The paraffin-embedded sections were deparaffinized in xylene and rehydrated.
Antigen retrieval was performed with protease K at 37 °C for 15 min. The
activity of endogenous peroxidase was blocked with a 3% H
Total RNA was isolated from L3-L5 DRGs (thereafter DRG) using the RNA-easy
Isolation Reagent (R701, Vazyme Biotech, Nanjing, China), and complementary DNA
(cDNA) was generated using a cDNA Synthesis Kit (DBI-2220, DBI®
Bioscience, Ludwigshafen, Germany). RT-qPCR was performed using a Quantitect SYBR
Green kit (DBI-2043, DBI® Bioscience) on a
StepOnePlus™ Real-Time PCR System (Applied
Biosystems®, Thermo Fisher Scientific, Waltham, MA, USA). The
following primers were used in the study: DCC sense:
5
The DRG lysates were centrifuged, and the supernatants were separated by sodium dodecyl sulfate polyacrylamide gel electrophoresis (SDS-PAGE) and blotted on polyvinylidene fluoride membranes (Pall Medical, Ann Arbor, MI, USA). The membranes were blocked with 5% milk in tris-buffered saline Tween-20 (TBST) at room temperature for 2 h and probed with primary antibodies overnight at 4 °C. The following primary antibodies were used: DCC (1:1000, ab273570; Abcam), UNC5B (1:1000, #13851; Cell Signaling Technology, Danvers, MA, USA), FAK (1:1000, #3285; Cell Signaling Technology), Phospho-FAK (Tyr397) (1:1000, #3283, Cell Signaling Technology), Rac1/Cdc42 (1:1000, #4651; Cell Signaling Technology), Phospho-Rac1/Cdc42 (Ser71) (1:1000, AF2393; Affinity Biosciences, Columbus, OH, USA) and GAPDH (1:2000, BL006B; Biosharp Life Sciences, Hefei, Anhui, China). The membranes were washed in TBST for 3 times and incubated for 1 h at room temperature with HRP-coupled anti-rabbit IgG secondary antibody (1:2000, #7074; Cell Signaling Technology). Finally, the membranes were identified using a computerized image analysis system (ChemiDoc XRS1, Bio-Rad, Hercules, CA, USA). The intensity of the protein bands was quantified using Image Lab software (ImageJ, Version 2.0.0-rc-69/1.52p, https://imagej.net/). The expression of target proteins was normalized against that of glyceraldehyde-3-phosphate dehydrogenase (GAPDH).
Three siRNAs were designed to knock down the expression of DCC (GenePharma,
Shanghai, China). Sequences of these siRNAs were: DCC-281-siRNA sense:
5
Rats were randomly divided into groups that received an intrathecal
administration of 2 µg DCC siRNA in 10 µL of in
vivo-jetPEI
Results of behavior tests are expressed as median
To elucidate the inoculation of Walker256 cells that resulted in pain behaviors and bone loss, pain behavior tests were performed on days 0, 4, 7, 11, 14, 17, and 21 (Fig. 1A). The paw withdrawal mechanical threshold (PWMT) and the limb use score for the BCP group decreased significantly following the disease progression (Fig. 1B,C). On day 21, the tibias of rats treated with BCP revealed apparent bone mass loss, whereas sham-treated rats exhibited no discernible bone loss (Fig. 1D, arrows). In the meantime, hematoxylin and eosin staining of the tibial sections showed notable osteolytic lesions with fractures along the cortical shaft of tibias from BCP rats (Fig. 1E, arrows). Moreover, the micro-CT images demonstrated a notable decline in bone mass in the tibias of BCP rats compared to the sham-treated rats. Additionally, a considerable decrease in the cortical bone volume/total volume (BV/TV) and the trabecular bone connectivity density (Conn D.) were verified through data analysis (Fig. 1F). These results indicate that the BCP model was established successfully.
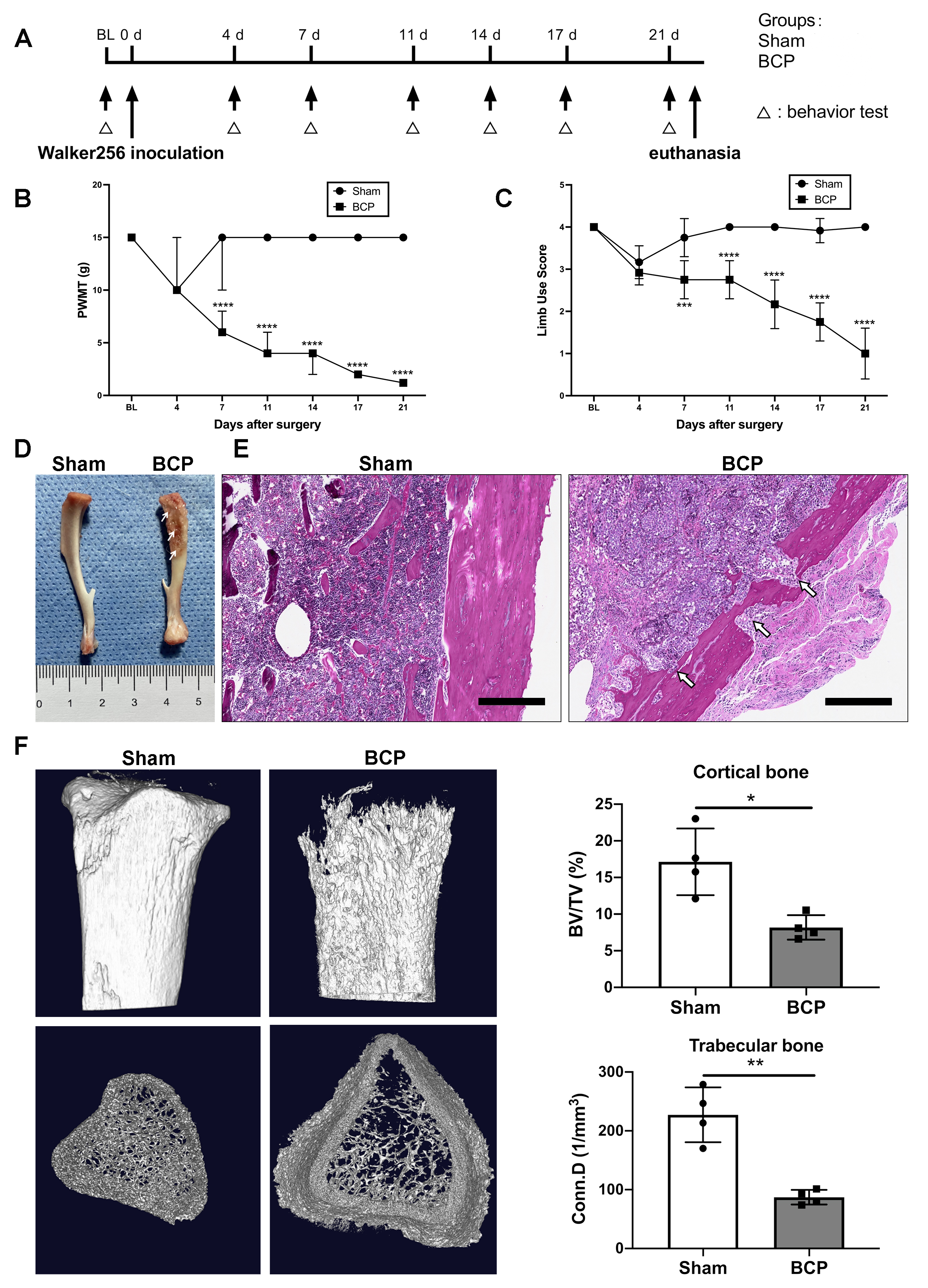
Intratibial inoculation of Walker256 mammary gland carcinoma
cells leads to pain behaviors and bone loss in rats. (A) Timeline of the
experimental schedule. (B) PWMT and (C) limb use score for pain behavior tests in
sham and BCP groups. n = 12 per group. Data are expressed as median
We examined the nociceptive nerve fibers that innervate the affected tibias by immunostaining CGRP. Compared to sham-treated rats, the innervation and density of CGRP + nociceptive nerve fibers increased in the tibias of rats with BCP (Fig. 2A,B). In addition, the immunohistochemistry staining revealed abnormal CGRP + nociceptive nerve fiber distribution (Fig. 2C, yellow arrows) near the trabecular bone surface. This was accompanied by significant multinuclear osteoclast activation, as confirmed by TRAP staining (Fig. 2C, blue arrows) in the ipsilateral tibias of BCP rats compared to sham-treated rats. These results suggest an increase in the innervation of CGRP + nociceptive nerve fibers in the affected tibias of BCP rats, which plays an important role in abnormal nociception during the development of hyperalgesia.
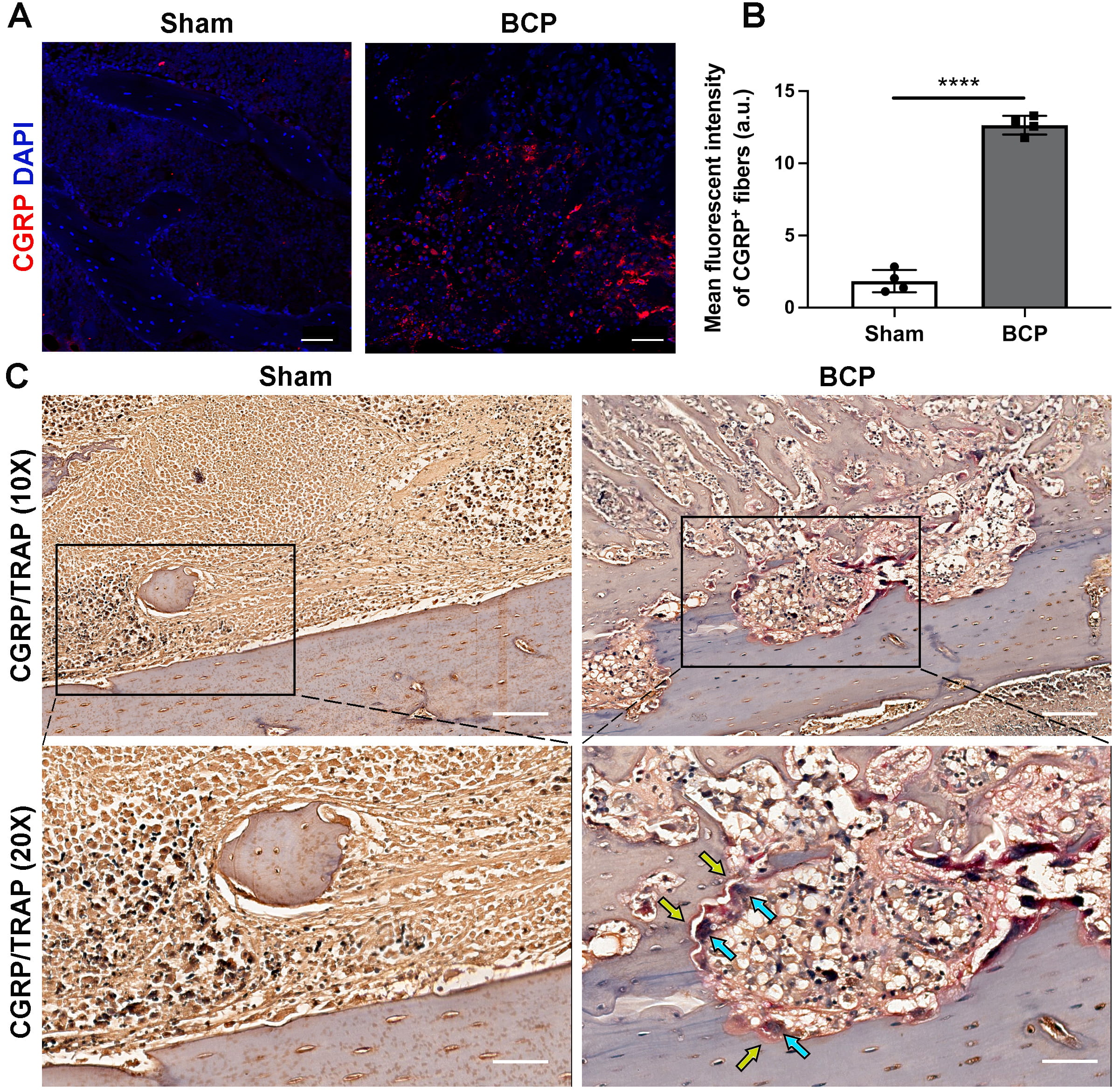
Innervation of CGRP + nociceptive nerve fibers increased in the
affected tibias during BCP progression. (A) Immunofluorescence analysis of CGRP
+ nociceptive nerve fibers (red) in the tibias on day 21 after Walker256 cell
inoculation. Scale bars, 50 µm. (B) Quantitative analysis of the mean
density of CGRP + sensory nerve fibers in panel A, n = 4 per group. (C)
Representative images of CGRP + nerve fibers (yellow arrows) and TRAP +
osteoclasts (blue arrows) staining in the tibias of sham-treated and BCP rats on
day 21, n = 4 per group. Scale bars, 200 µm (10
Recently, Zhu et al. [22] demonstrated that osteoclast-derived netrin-1 is important in regulating osteoarthritis pain. It is known that activated osteoclasts in the microenvironment of bone metastasis are critical for the initiation and development of BCP [1, 35]. Consistent with this evidence, we detected activation of osteoclasts by TRAP staining (blue arrows) along with upregulation of netrin-1 (yellow arrows) in the affected tibias of BCP rats (Fig. 3A). Meanwhile, immunofluorescent staining showed prominent upregulation of netrin-1 expression in the affected tibias of BCP rats compared with sham-treated rats (Fig. 3B,C). Furthermore, double immunofluorescent staining showed spatial proximity of netrin-1 and CGRP expressions in the affected tibias of BCP rats (Fig. 3B). These findings imply that in the metastatic bone lesions of BCP rats, netrin-1 might be secreted by differentiated osteoclasts, resulting in the heightened innervation of CGRP + nociceptive nerve fibers.
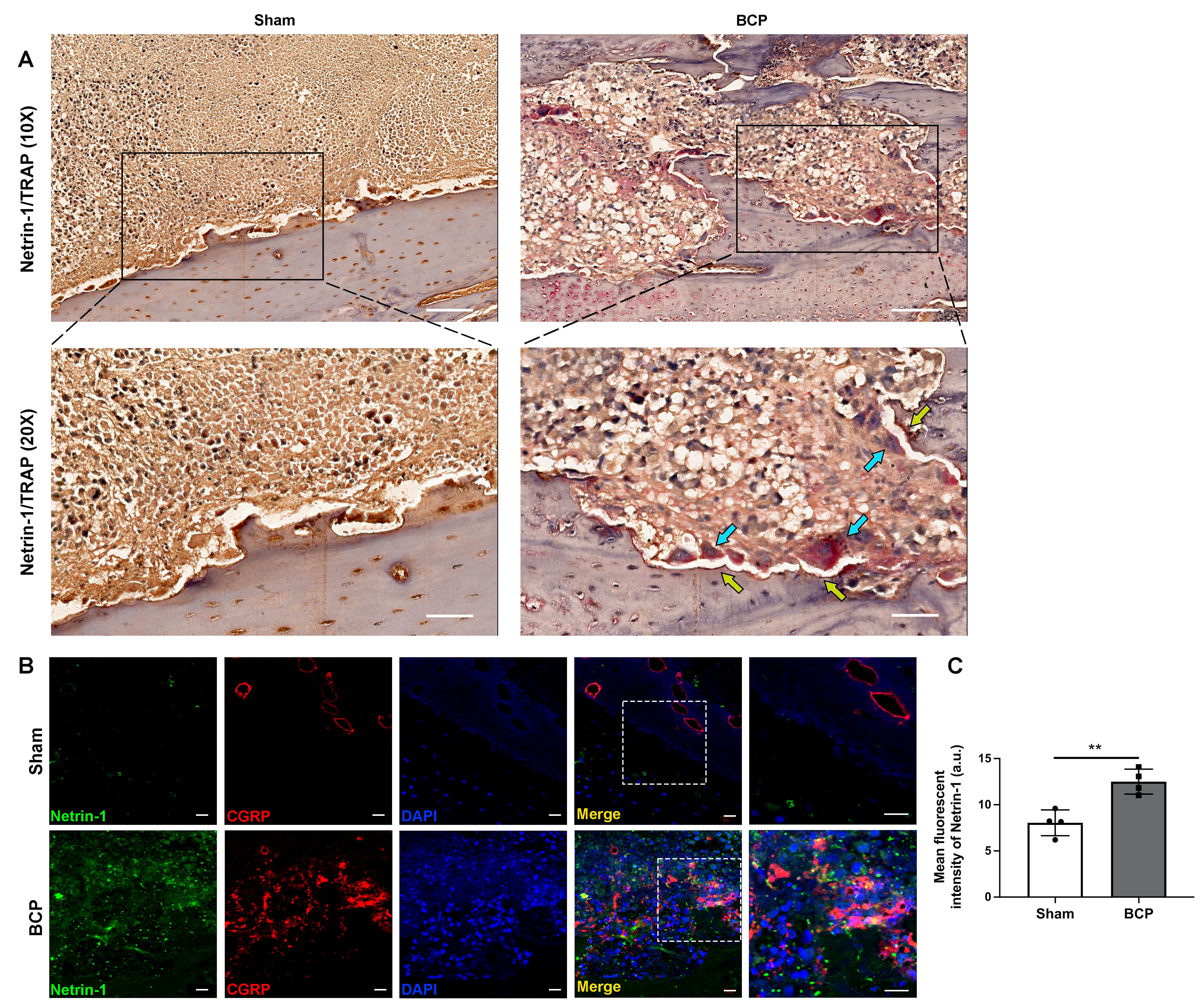
Increased osteoclast-derived netrin-1 was related to the
sprouting of nociceptive nerve fibers in the tibias of BCP rats. (A)
Representative images of immunohistochemistry staining of netrin-1 (yellow
arrows) and TRAP + osteoclasts (blue arrows) in the affected tibias of
sham-treated and BCP rats on day 21, n = 4 per group. Scale bars, 200 µm
(10
We examined the expressions of the receptors of netrin-1 in the ipsilateral DRG, and the results demonstrated that the transcription of DCC mRNA (Fig. 4A), rather than that of UNC5B mRNA (Fig. 4B), was significantly upregulated in BCP rats compared to sham-treated rats. Consistently, western blotting showed upregulation of DCC expression in the DRG of BCP rats. In contrast, the expression of UNC5B was not significantly altered (Fig. 4C). In addition, downstream signals of netrin-1/DCC signaling were further verified by examining the phosphorylation levels of FAK and Rac1/Cdc42 in BCP rats. The results indicated that phosphorylation of FAK and following phosphorylation of Rac1/Cdc42 were significantly upregulated in BCP rats compared to the sham-treated group (Fig. 4C). Moreover, immunofluorescent staining showed prominent upregulation of DCC expression in the affected tibias of BCP rats compared with sham-treated rats (Fig. 4D,E). Double immunofluorescent staining showed evident co-localization of DCC and CGRP in the affected tibias of BCP rats (Fig. 4D). The findings suggest that netrin-1 may control nerve regeneration through the DCC receptor and its associated downstream signals, promoting the development of BCP.
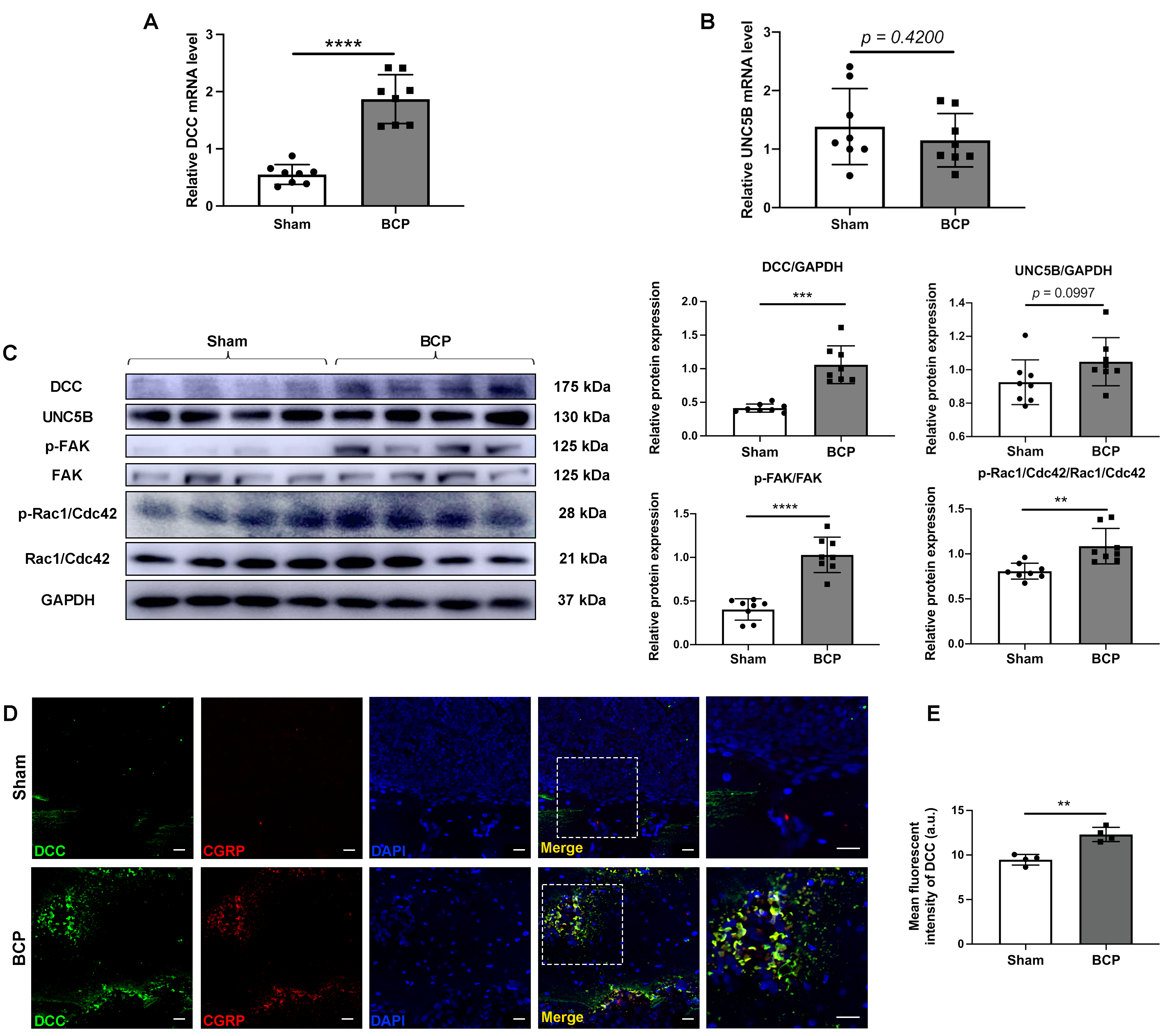
Netrin-1 contributed to the sprouting of sensory nerve fiber in
the metastatic bone lesions through the netrin-1/DCC signaling pathway. RT-qPCR
analysis of the expression of (A) DCC and (B) UNC5B in the DRG on day 21. n = 8
per group. (C) The expressions of DCC, UNC5B, and phosphorylation levels of FAK
and Rac1/Cdc42 in the DRG of rats in the sham and BCP groups on day 21 were
determined by western blotting with representative protein bands presented. GAPDH
was detected as an internal control. n = 8 per group. (D) Double
immunofluorescence of DCC and CGRP in the affected tibias of sham-treated and BCP
rats on day 21. Scale bars: 20 µm. (E) Quantitative analysis of the mean
density of DCC. n = 4 per group. Data are expressed as mean
To further elucidate the role of activated netrin-1/DCC signaling during BCP hypersensitivity, DCC was knocked down by siRNA in BCP rats in vivo. DCC siRNA or vehicle were intrathecally injected on days 10, 13, and 16 after BCP model establishment. Pain behavior tests were conducted immediately prior to the start of the experiment, on the first day of treatment, and then daily during the treatment period (Fig. 5A). Targeting by siRNA significantly reduced DCC mRNA transcription (Supplementary Fig. 1) and inhibited the downstream phosphorylation levels of FAK and Rac1/Cdc42 (Fig. 5B). Meanwhile, we detected a significantly decreased innervation of CGRP + nociceptive nerve fibers in the affected tibias (Fig. 5C,D). As anticipated, the rats exhibited a reduction in mechanical pain-related behaviors, as evidenced by the enhancement of PWMT levels and limb utilization (Fig. 5E,F), from day 11 to day 17 following intrathecal siRNA administration. These findings demonstrate that DCC signaling is essential in controlling peripheral nociceptive nerve innervation and plays a crucial role in developing and maintaining bone cancer pain.
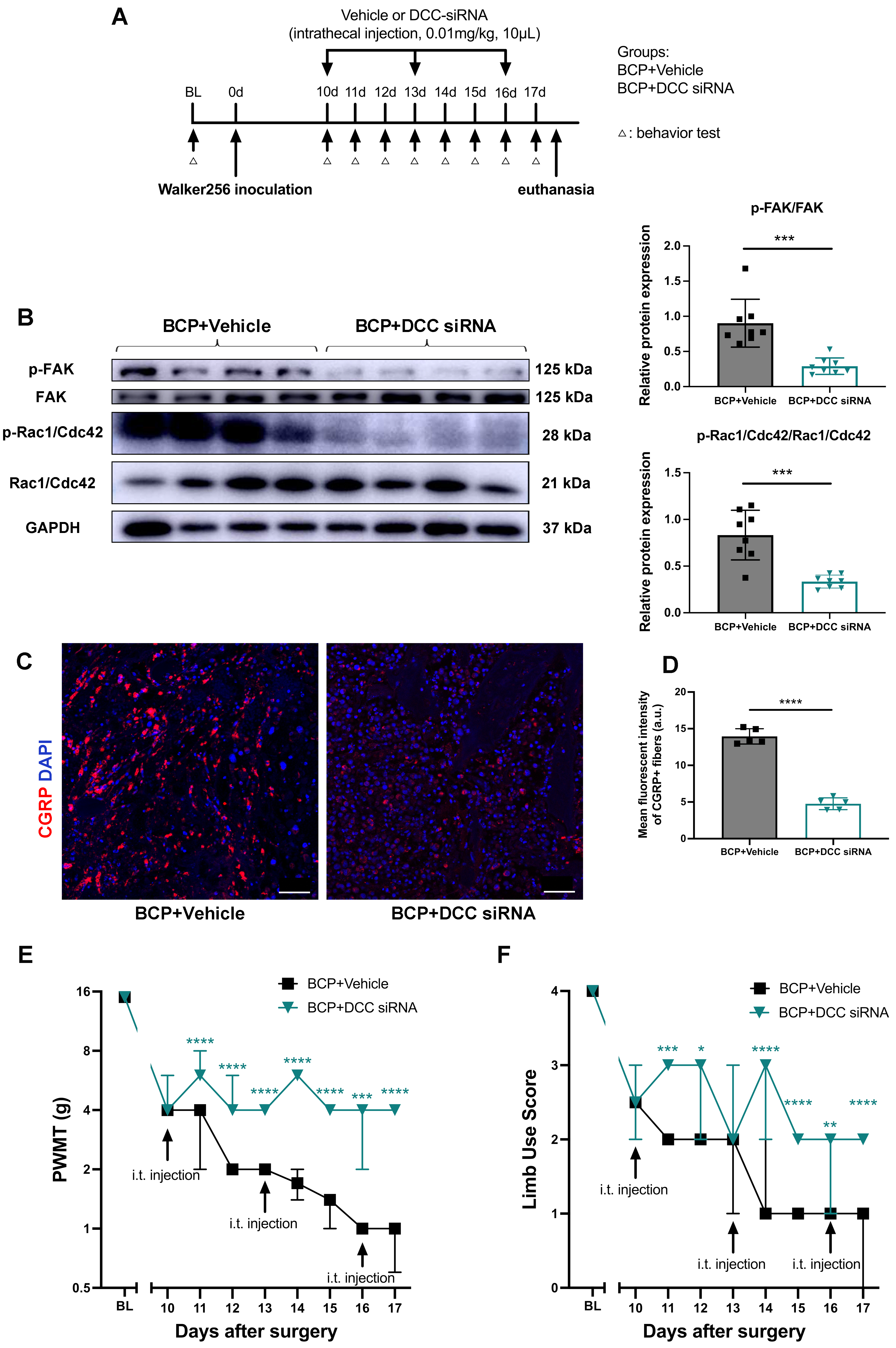
Targeting DCC by intrathecal siRNAs administration in BCP rats.
(A) Timeline of the experimental schedule for siRNA or vehicle injections. (B)
Expressions of p-FAK/FAK and p-Rac1/Cdc42/Rac1/Cdc42 in the DRG of BCP rats
treated with intrathecal vehicle or DCC-siRNA administration were determined by
western blotting with representative protein bands presented. GAPDH was detected
as an internal control. n = 8 per group. Data are expressed as mean
The present study has identified for the first time the essential role of netrin-1 in mediating BCP by increasing the innervation of peripheral CGRP + nociceptive nerve fibers in BCP rats through the netrin-1/DCC signaling pathway. Specifically, we observed elevated innervation of CGRP + nociceptive nerve fibers in the affected tibias of BCP rats. Activated osteoclasts and netrin-1 expression increased in the metastatic bone lesions, demonstrating a spatial correlation with the heightened presence of CGRP + nociceptive nerve fibers. Conversely, the DRG of BCP rats displayed elevated expressions of DCC and its downstream signals. Furthermore, DCC expression in the metastatic site was also enhanced and colocalized with the increased CGRP + nerve fibers. Notably, the intrathecal administration of DCC siRNA yielded a significant reduction in downstream signal expressions, peripheral CGRP + nociceptive nerve innervation, and ultimately alleviated pain behaviors in BCP rats (Fig. 6).
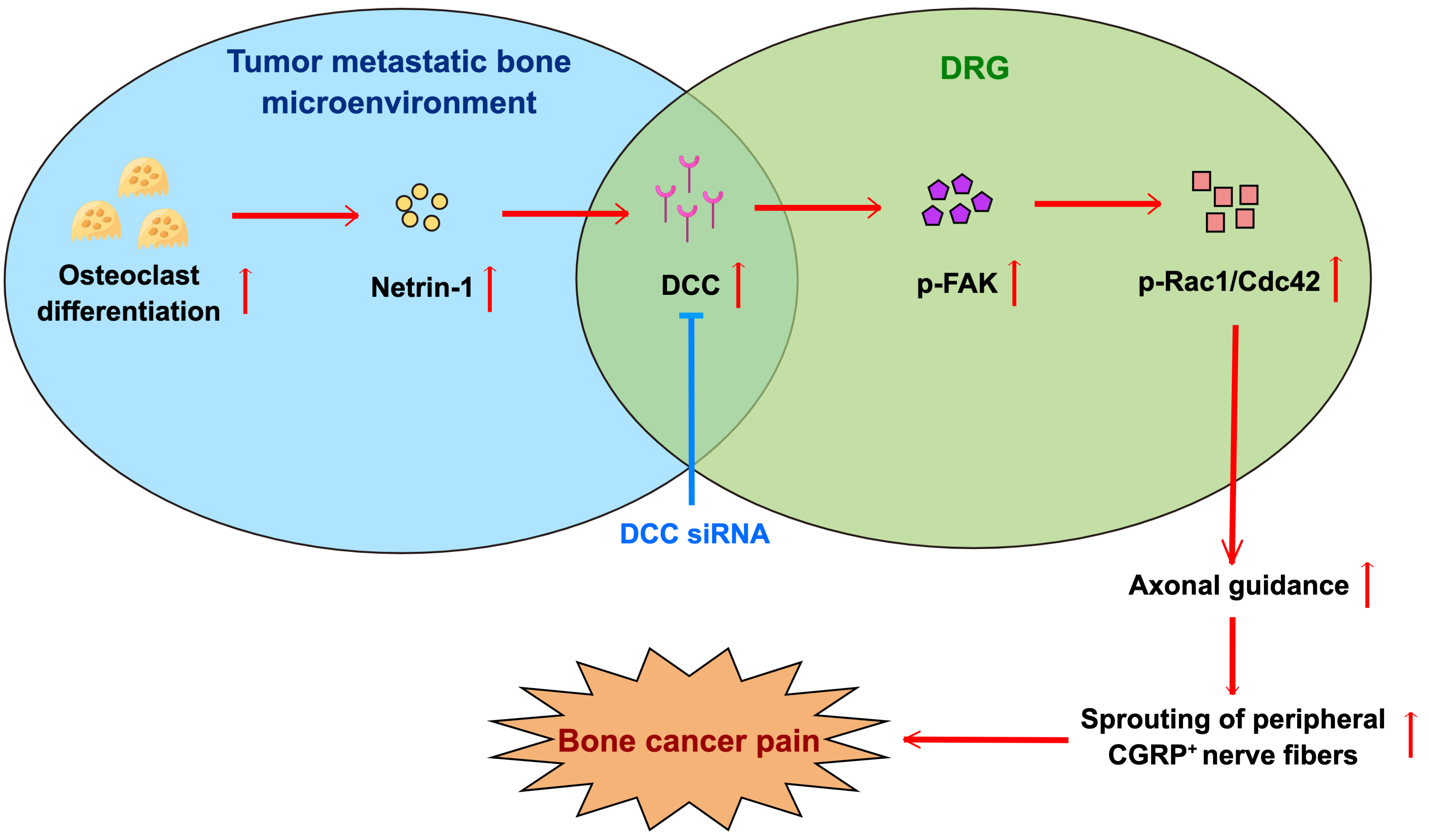
Schematic summary of the mechanism by which netrin-1/DCC signaling aggravates bone cancer pain. Expression of netrin-1 in the metastatic bone lesion was significantly increased, subsequently activating netrin-1/DCC/FAK/Rac1/Cdc42 signaling pathway to promote its biofunction on axonal guidance, thus promoting the innervation of peripheral CGRP + nerve fibers and eventually aggravated bone cancer pain. Intrathecal injection of DCC siRNA blocked the signal transduction of DCC signaling and was efficacious in alleviating bone cancer pain. This figure was created on Figdraw (https://www.figdraw.com/#/).
The initiation of BCP is a complex process. Sprouting of nociceptive nerve fibers is believed to be closely related to BCP development [36, 37, 38, 39, 40]. Following injury or disease, various factors and axon guidance molecules released by stromal and inflammatory cells can potentially induce the sprouting of peripheral nociceptive nerve fibers, resulting in heightened sensitivity of the metastatic bone lesion, thereby exacerbating bone cancer pain. Furthermore, our previous investigation demonstrated that excessive activation of bone morphogenetic protein-2 (BMP2) represents a crucial step in the mechanism of peripheral sensitization of bone cancer pain [41]. On the other hand, BMP2 is believed to be closely related to early axonal regrowth after nerve injury [42, 43]. Overall, this evidence shows that controlling bone remodeling and nociceptive nerve innervation is crucial for the occurrence and development of pain sensation during bone metastases of tumors. Recently, studies have confirmed the importance of netrin-1, one of the direct modulators of neurite elongation, in modulating pain development by regulating neurite elongation [22].
In the present study, netrin-1 expression in the affected bone of BCP rats was higher than that in the sham group. In BCP development, the netrin-1 receptor DCC is upregulated in the ipsilateral DRG rather than UNC5B. CGRP is spatially proximal to netrin-1 in BCP rats and colocalized with DCC in the affected tibias. Targeting DCC through intrathecal injection of siRNA significantly reduced the innervation of CGRP + nociceptive nerve fibers in the affected bone and improved pain behaviors in BCP rats.
Moreover, multiple studies have reported that focal adhesion kinase (FAK), a subtype of protein tyrosine kinases (PTKs), is activated by netrin-1/DCC signaling in the neurons of the corpus callosum, which plays an important part in axonal formation and elongation [44, 45, 46, 47]. Recently, Ye et al. [48] reported that netrin-1 activates the cytoskeletal regulator Rac1/Cdc42 and downstream kinase Pak1 by binding to DCC, thereby inducing growth cone expansion and neurite elongation. There was a significant increase in p-FAK and p-Rac1/Cdc42 in the ipsilateral DRG of BCP rats. Intrathecal injection of DCC-siRNA notably inhibited the phosphorylation levels of FAK and Rac1/Cdc42. In line with this evidence, the current study identified the potential role of netrin-1 in the BCP development by inducing abnormal sprouting of nociceptive CGRP + neurite through netrin-1/DCC and its downstream FAK/Rac1/Cdc42 signaling pathway.
As reported, macrophage-derived netrin-1 is a crucial factor that promotes neurite growth and sensitization through the upregulation of CGRP in endometriosis [26]. Additionally, senescent fibroblast cell-secreted netrin-1 has been demonstrated to significantly modulate aging-related diseases by recruiting sympathetic fibers [27]. Last, netrin-1 secreted by osteoclasts in osteoarthritis is convinced to participate in augmented sensory innervation and osteoarthritis pain [22]. The enrichment of netrin-1 in various locations is crucial for neurite elongation and body function modification. In our study, we observed significant upregulation of osteoclast-derived netrin-1 only in affected bone tissues of BCP rat models. However, whether other sources of netrin-1 synthesis and secretion are associated with BCP progression still needs to be confirmed.
There are several limitations to this study. One aspect to consider is that all experiments were conducted using female rats due to the utilization of Walker256 breast cancer cells to establish the BCP model. Existing literature indicates that sex hormones, such as estrogen or testosterone, may influence pain phenotypes [49, 50]. To mitigate gender-related bias, we opted to use female rats in this study exclusively. However, further research is warranted to explore the role of netrin-1/DCC signaling in the development of BCP in other gender, species, or BCP models established using different cell lines, such as MDAMB231 cancer cells or SHZ-88 cancer cells. Furthermore, recent investigations have indicated that the netrin-1/DCC signaling pathway positively impacts peripheral nerve regeneration [51]. Therefore, when considering the therapeutic potential of netrin-1 and its downstream signaling in managing BCP, it is crucial to account for its dual functionality.
Moreover, netrin-1 is reported to be associated with inhibiting tumor growth and metastasis through netrin-1/UNC5B signaling [52]. Whether upregulated netrin-1 in the metastatic bone microenvironment ameliorates BCP through this mechanism needs further investigation. As for the methodology, additional applications of other technologies and devices, such as electrophysiological recording, dynamic weight-bearing (DWB) systems, and other behavioral tests, may help identify different pain phenotypes [53]. Lastly, BCP is a complex complication of advanced metastatic bone cancer with intricate and complicated mechanisms. Netrin-1/DCC signaling is a potential therapeutic target for BCP. More molecules and signaling pathways, such as GFAP, IBA-1, or glutamate, need to be confirmed to provide new insights for BCP management.
Despite the limitations discussed above and given the increasing incidence of bone metastases of tumors and the inadequate control of BCP by existing available medication, a better understanding of BCP mechanisms would potentially help discover more effective analgesics. Our study has determined that netrin-1/DCC signaling is essential in remodeling nociceptive nerve innervation of metastatic bone lesions during BCP development. Inhibition of netrin-1/DCC signaling reduces innervation of nociceptive nerve fibers and ameliorates pain behaviors at the onset of bone metastasis of tumors; therefore, it may have therapeutic potential for BCP management.
The findings highlighted for the first time that the activation of netrin-1/DCC signaling may contribute to bone cancer pain in rats by increasing the innervation of peripheral CGRP + nociceptive nerve fibers. These observations may provide a novel strategy for studying and managing bone cancer pain.
The datasets used and/or analyzed during the current study are available from the corresponding author on reasonable request.
ZG, WW, XY and JW designed the research study. ZG, YZ, XL and KW performed the research. JW and XY gave experimental technical guidance. ZG and YZ analyzed the data. ZG wrote the manuscript. All authors contributed to editorial changes in the manuscript. All authors read and approved the final manuscript. All authors have participated sufficiently in the work and agreed to be accountable for all aspects of the work.
All experiments were performed in accordance with protocols approved by the Animal Care and Use Committee of Shanghai Chest Hospital, Shanghai Jiao Tong University, School of Medicine [Permission No. KS (Y)20186].
The authors would like to express their gratitude to EditSprings for the expert linguistic services provided.
This work was supported by the National Natural Science Foundation of China (82071233) and the Shanghai Sailing Program (21YF1442900).
The authors declare no conflict of interest.
Publisher’s Note: IMR Press stays neutral with regard to jurisdictional claims in published maps and institutional affiliations.