- Academic Editor
Background: Glaucoma patients frequently present with depressive symptoms, the development of which is closely associated with amygdalar activity. However, no studies to date have documented glaucoma-related changes in the functional connectivity (FC) of the amygdala. Accordingly, resting-state functional magnetic resonance imaging (rs-fMRI) analyses were herein used to evaluate changes in amygdalar FC in primary angle-closure glaucoma (PACG) patients. Methods: In total, this study enrolled 36 PACG patients and 33 healthy controls (HCs). Complete eye exams were conducted for all PACG patients. After the preprocessing of magnetic resonance imaging (MRI) data, the bilateral amygdala was selected as a seed point, followed by the comparison of resting-state FC between the PACG and HC groups. Then, those brain regions exhibiting significant differences between these groups were identified, and relationships between the FC coefficient values for these regions and clinical variables of interest were assessed. Results: These analyses revealed that as compared to HC individuals, PACG patients exhibited reductions in FC between the amygdala and the cerebellum_8, vermis_4_5, anterior central gyrus, supplementary motor area, paracentral lobule, putamen, middle frontal gyrus, and posterior cingulate gyrus, while enhanced FC was detected between the right and left amygdala. No significant correlations between these changes in amygdalar any any disease-related clinical parameters or disease duration were noted. Conclusions: Patients with PACG exhibit extensive resting state abnormalities with respect to the FC between the amygdala and other regions of the brain, suggesting that dysregulated amygdalar FC may play a role in the pathophysiology of PACG.
Glaucoma refers to a series of ocular diseases characterized by progressive optic nerve head (ONH) degeneration retinal ganglion cell (RGC) loss, and concomitant defects of the visual field (VF) [1]. Glaucoma is the leading global cause of irreversible blindness, with an estimated 76 million patients throughout the world and a projected increase in this number to 112 million as of 2040 [2]. Glaucoma causes the blindness of 5.1–6.8 million individuals each year, often resulting in bilateral blindness that causes extensive harm to individuals, their families, and societies [3]. Glaucoma primarily causes selective RGC death within the human retina, with RGC dendrites comprising the optic nerve and providing the majority of the inputs to the primary visual cortex. As RGC viability declines, optic dendrite counts thus decrease, leading to a drop in sensory input to the primary motor cortex and a concomitant drop in functional connectivity (FC) to the secondary visual cortex. As such, the damage associated with glaucoma may extend beyond ONH or RGC degeneration, causing the deterioration of the optic nerve, visual cortex, and potentially even certain non-visual areas of the brain [4, 5]. In light of this evidence, some scholars have suggested regarding glaucoma as a form of neurodegenerative disease [6].
In most prior magnetic resonance imaging (MRI) studies, primary open-angle glaucoma (POAG) patients have been the primary focus. However, the most common glaucoma subtype in East Asia and other portions of Asia is primary angle-closure glaucoma (PACG) [7], and blindness rates in these regions are higher than those in Western nations [8]. Resting-state functional magnetic resonance imaging (rs-fMRI) has emerged as an invaluable tool for studies of intrinsic brain activity in glaucoma patients, offering key insights into the pathological basis for different conditions. In PACG patients, recent studies have revealed abnormal regional homogeneity (ReHo) [9] and low-frequency fluctuation amplitude (ALFF) [10] in several regions of the brain. Cai et al. [11] observed compensatory increases in the degree centrality (DC) values of the bilateral visual cortex and left anterior central gyrus following surgery in patients with PACG, while Chen et al. [12]determined that short- and long-range functional connectivity density spatial distributions differed in PACG patients. Wang et al. [13] observed extensive resting-state FC (rs-FC) abnormalities between the thalamus and other brain regions in individuals with PACG. In another report, changes in information interaction and integration between cerebral hemispheres have been detected in PACG patients, suggesting that this condition may be associated with the impaired interaction and integration of visual information [14]. These reports offer a range of perspectives on PACG-associated characteristics and spontaneous activity changes.
In addition to the ophthalmic changes characteristic of glaucoma, a growing number of studies have indicated that affected patients experience higher rates of a range of other debilitating symptoms including depression and anxiety [15, 16, 17]. Indeed, studies of a large population have revealed a significant correlation between glaucoma and anxiety/depression [18, 19]. Relative to patients with POAG, PACG patients reportedly experience more serious symptoms of depression [20]. The amygdala has been found to serve as an important regulator of emotional activity [21], with MRI scanning having revealed depression-related decreases in amygdalar volume [22]. Limited studies have also explored the neuroimaging basis for psychiatric symptoms in glaucoma patients. One 3T MRI study, for example, found that reductions in left amygdalar volume in POAG patients were related to changes in mood [23]. At present, however, there remains relatively little neuroimaging research focused on psychiatric symptoms in patients with PACG. However, amygdalar abnormalities may be involved in the incidence of depression in PACG patients, highlighting a need for further research on this topic.
In light of the above research findings, the present study sought to employ rs-FC measurements as an approach to detecting abnormal amygdalar FC in patients with PACG. These studies were conducted based on the hypothesis that PACG patients exhibit changes in FC between the amygdala and other regions of the brain, and that these changes are associated with PACG-related clinical parameters.
In total, 40 patients with PACG hospitalized in the First Affiliated Hospital of
Nanchang University were recruited for this study. Eligibility criteria for PACG
patients included: (1) gonioscopy- or slit-lamp-confirmed binocular angle
stenosis or closure; (2) visual field defects characteristic of PACG, including
paracentral scotoma, nasal staircase, and/or a tubular visual field; (3) evidence
of optic disc depression on fundus examination; (4) right-handedness; and (5) no
history of drug treatment or surgery to address this condition. PACG patients
were excluded if they: (1) exhibited other ocular diseases; (2) were affected by
diabetes, hypertension, or other underlying diseases; (3) had any history of
surgery to treat glaucoma; (4) exhibited other nervous system or
neurodegenerative diseases; (5) presented with incomplete clinical or MRI data;
or (6) exhibited maximum head displacement in any direction (x, y, z) during
rs-fMRI scanning greater than 2.5 mm or head angular rotation exceeding
2.5°. Based on these criteria, 36 PACG patients (21 female, 15 male)
were enrolled following the exclusion of 4 individuals exhibiting
In addition, 33 age-, gender-, and education level-matched right-handed healthy control (HC) subjects were recruited (17 female, 16 male). HCs were excluded if they: (1) exhibited any ocular or systemic diseases; (2) were diagnosed with diabetes, hypertension, or other underlying conditions; (3) had any neurological or neurodegenerative diseases; (4) exhibited MRI contraindications such as claustrophobia or pacemaker installation; or (5) were not cooperative or exhibited maximum head displacement in any direction (x, y, z) during rs-fMRI scanning greater than 2.5 mm or head angular rotation exceeding 2.5°.
The Declaration of Helsinki was observed when conducting this study, which received approval from the Human Research Ethics Committee of the First Affiliated Hospital of Nanchang University (NO.2022 (3-008)). All participants provided written informed consent. Participant characteristics are summarized in Table 1.
Condition | PACG | HC | p-value |
Age (years) | 54.78 |
54.27 |
0.796 |
Gender (male/female) | 15/21 | 16/17 | 0.282 |
Duration of PACG (days) (range) | 2–3650 | - | N/A |
IOP (mmHg) | 28.64 |
- | N/A |
RNFLT (µm) | 76.78 |
- | N/A |
A-C/D | 0.67 |
- | N/A |
V-C/D | 0.65 |
- | N/A |
Mean VA (range) | 0.52 |
- | N/A |
Head motion | 0.09 |
0.11 |
0.208 |
PACG, primary angle-closure glaucoma; HC, healthy control; IOP, RNFLT, A-C/D, V-C/D, and VA are given as binocular means. VA, visual acuity; IOP, intraocular pressure; A-C/D, average cup-to-disc ratio; V-C/D, vertical cup-to-disc ratio; RNFLT, retinal nerve fiber layer thickness; N/A, not applicable.
All rs-fMRI data used to conduct this study were collected with a Siemens
Magnetom Skyra 3.0T magnetic resonance scanner (Siemens, Erlangen, Germany) in
the Department of Radiology, of the First Affiliated Hospital of Nanchang
University, China. Before undergoing MRI scanning, study participants were
directed to sit for 10 minutes. During scanning, participants were directed to
remain relaxed with their eyes closed while not thinking about anything specific.
Head movement and noise mitigation were achieved with bilateral sponge cushions,
and scanning was performed in the head-advanced supine position. Scans entailed
the collection of 240 volumes over 8 min using the following settings: repetition
time (TR) = 2000 ms; echo time (TE) = 40 ms; Flip Angle = 90°; Field of
view = 240 mm
The MRIcro (V1.0, University of Pittsburgh, Pittsburgh, PA, USA) program was used to check all rs-fMRI data, discarding any data of
poor quality, after which the Brain Imaging Data Processing and Analysis (DPABI)
toolbox [24] was used to preprocess these data in MATLAB 2018b (MathWorks,
Natick, MA, USA). Preprocessing steps included: (1) the removal of the first 10
time points; (2) the correction of the remaining 230 volumes for 3D head motion
and slice time; (3) the spatial normalization of all functional data to the
Montreal Neurological Institute (MNI) template with subsequent 3
The bilateral amygdala region in the automated anatomical labeling (AAL) template of the WFU_PickAtlas program was initially selected as the region of interest (ROI) for FC analyses, extracting the time series of all voxels for that ROI. Then Pearson correlation analyses between this voxel time series and the time series of all other voxels in the whole brain in order to generate a map of FC correlation coefficients. The resultant values were then subjected to Fisher Z transformation to improve consistency with the normal distribution so as to permit statistical analyses.
All PACG patients completed a comprehensive ophthalmologic examination. Slit-lamp examination and gonioscopy were used to assess anterior chamber angle status, while a tonometer was used to measure intraocular pressure (IOP). Optical coherence tomography (Cirrus HD-OCT) (Heidelberg Engineering, Heidelberg, Baden-Württemberg, Germany) was also employed to assess retinal nerve fiber layer thickness (RNFLT), average cup-to-disc ratio (A-C/D), and vertical cup-to-disc ratio (VC/D) values. Visual acuity (VA) and disease course were also recorded.
Demographic and clinical data were analyzed using the Statistical Package for the Social Sciences (SPSS) version 24.0 (Chicago, IL, United States). Age and clinical parameters were compared with Student’s t-tests, whereas gender distributions were compared between groups using chi-square tests.
Two-sample t-tests were used to compare FC between each ROI and the
whole brain voxel, using age, gender, and other variables as covariates.
p
Pearson correlation analyses were used to examine the association between
altered amygdalar FC in PACG patients and clinical parameters including disease
duration, RNFLT, A-C/D, V-C/D, and IOP. These analyses were completed with SPSS
24.0, with larger absolute values (range: –1 to 1) being indicative of stronger
correlations. p
PACG patients enrolled in this study were 54.78
Initially, whole brain rs-FC was compared between PACG and HC subjects based on the voxel-based bilateral amygdala seeds (Table 2). Relative to HC individuals, PACG patients exhibited reduced functional connections between the right amygdala and the left cerebellum_8, the vermis_4_5, the bilateral precentral gyrus (PreCG), the right supplementary motor area (SMA), and the left paracentral lobule (PCL), whereas enhanced connectivity with the contralateral amygdala was detected in these same patients. These patients also presented with reduced functional connections between the left amygdala and the bilateral cerebellum_8, the vermis_4_5, the bilateral putamen, the left middle frontal gyrus (MFG), the left posterior cingulate gyrus (PCG), and the left PreCG (Figs. 1,2,3,4).
Seed-ROIs | L/R | Brain area | Voxel | MNI coordinates of peak voxel | t-value | ||
X | Y | Z | |||||
Right amygdala | |||||||
L | Cerebellum_8 | 65 | –21 | –54 | –45 | –5.4326 | |
Vermis_4_5 | 59 | 3 | –60 | –30 | –6.1058 | ||
L | Amygdala | 48 | –24 | –3 | –18 | 20.6521 | |
L | Precentral | 93 | –39 | –9 | 42 | –5.2901 | |
R | Precentral | 77 | 21 | –9 | 63 | –4.4531 | |
R | Supp_Motor_Area | 76 | 12 | 12 | 60 | –5.3577 | |
L | Paracentral_Lobule | 37 | –15 | –18 | 69 | –5.2054 | |
Left amygdala | |||||||
L | Cerebellum_8 | 69 | –18 | –54 | –48 | –5.0134 | |
R | Cerebellum_8 | 26 | 39 | –42 | –48 | –4.2911 | |
Vermis_4_5 | 55 | –3 | –63 | –30 | –5.4597 | ||
L | Putamen | 107 | –30 | –6 | 9 | –5.2192 | |
R | Putamen | 105 | 30 | 6 | 9 | –5.5938 | |
L | Cingulum_Post | 27 | –9 | –48 | 21 | –4.8396 | |
L | Frontal_Mid | 28 | –24 | 45 | 21 | –4.0207 | |
L | Precentral | 317 | –24 | 12 | 57 | –5.5461 |
Voxel level p
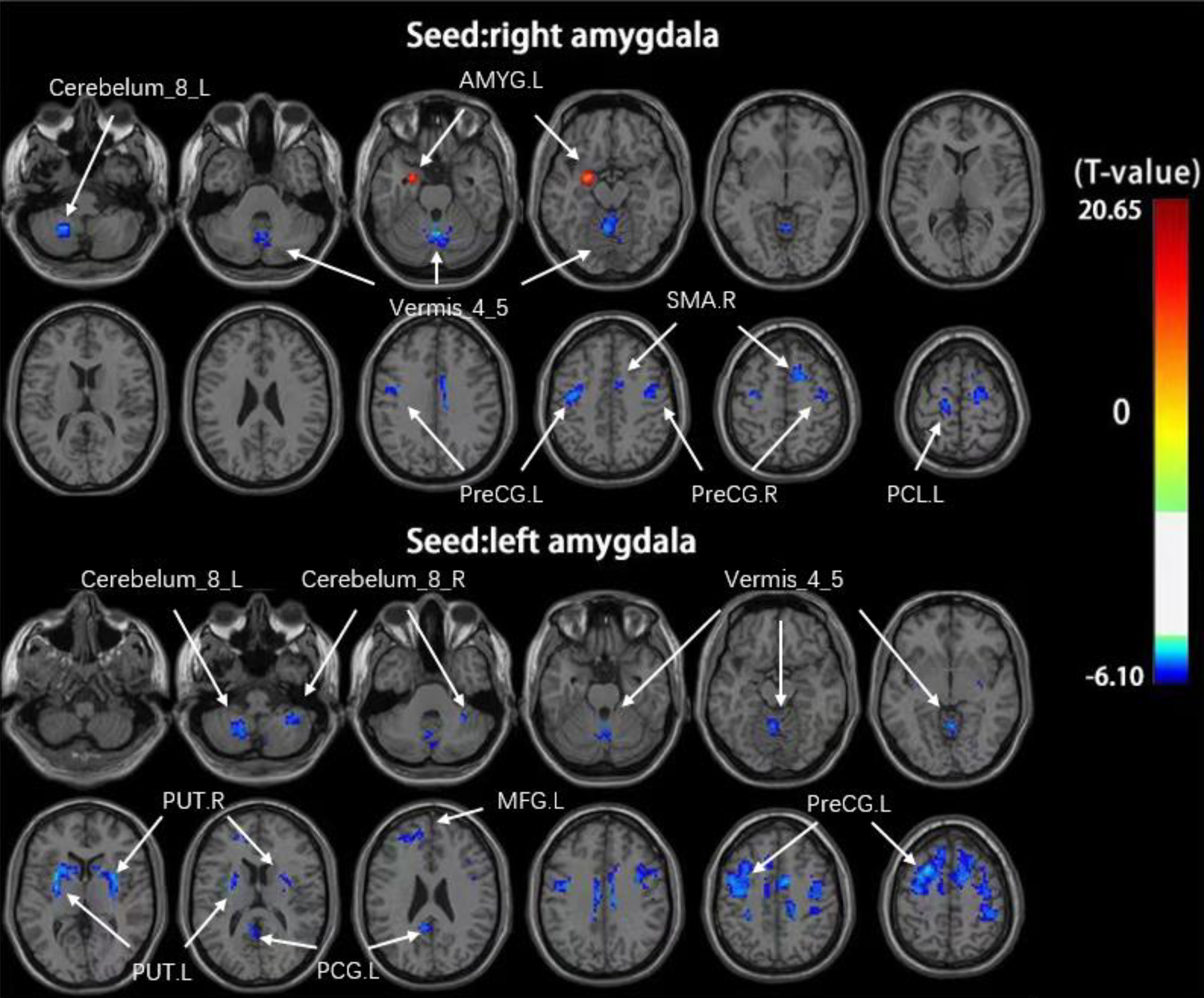
Regions of the brain exhibiting significantly altered rs-FC for
the bilateral amygdala (voxel-level p
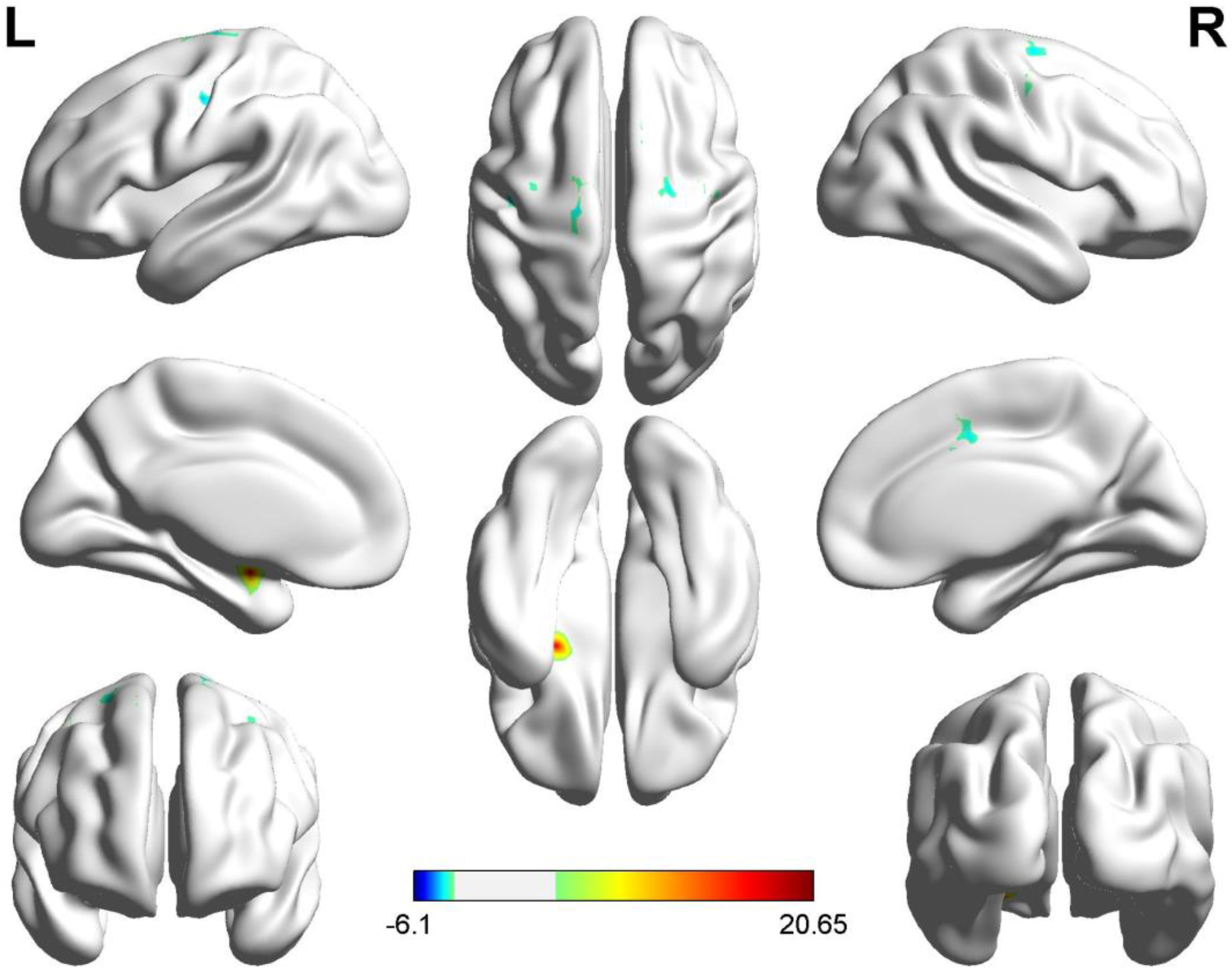
Voxelwise FC comparisons between PACG patients and HC based on
the right amygdala seed (voxel-level p
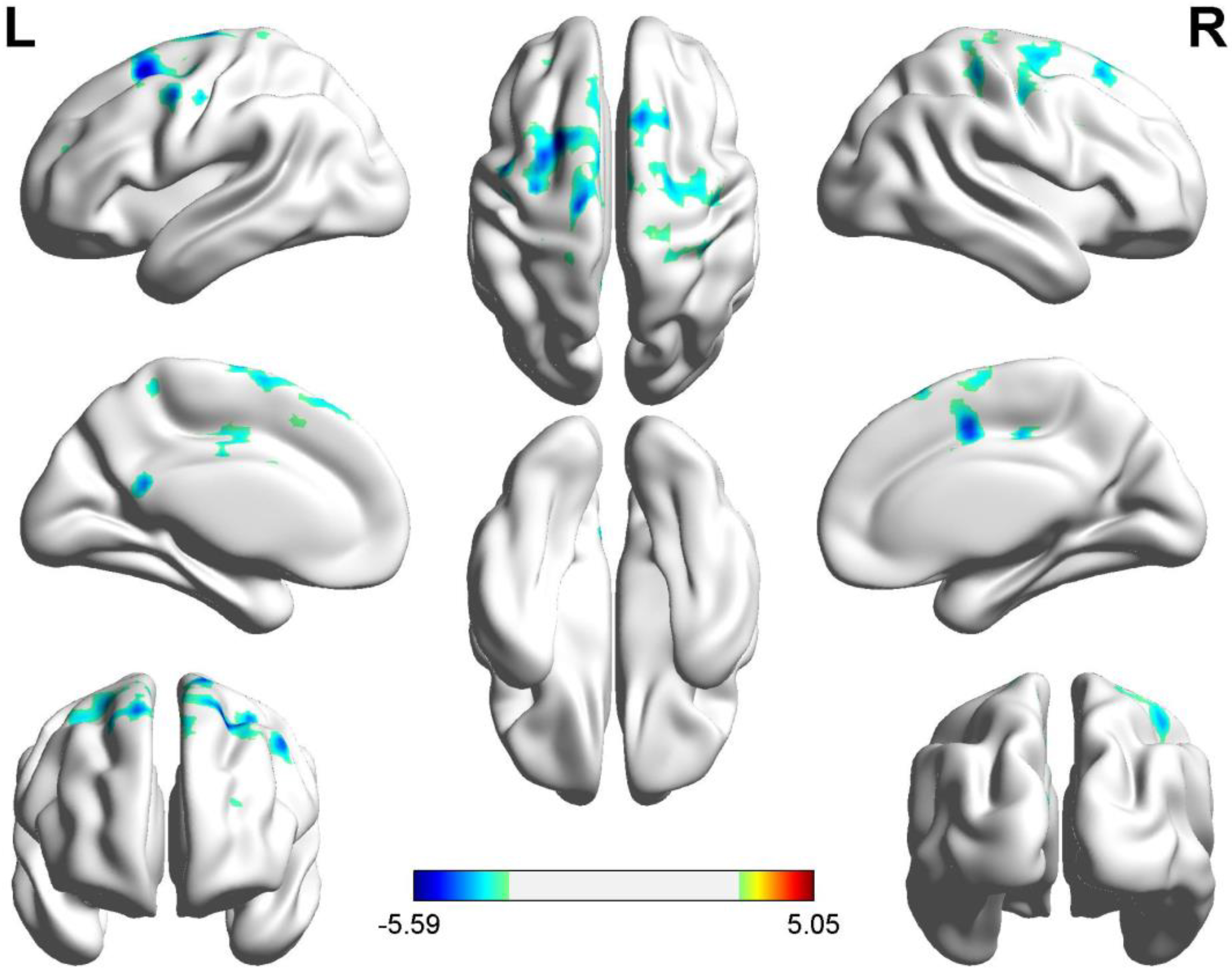
Voxelwise FC comparisons between PACG patients and HC based on
the left amygdala seed (voxel-level p
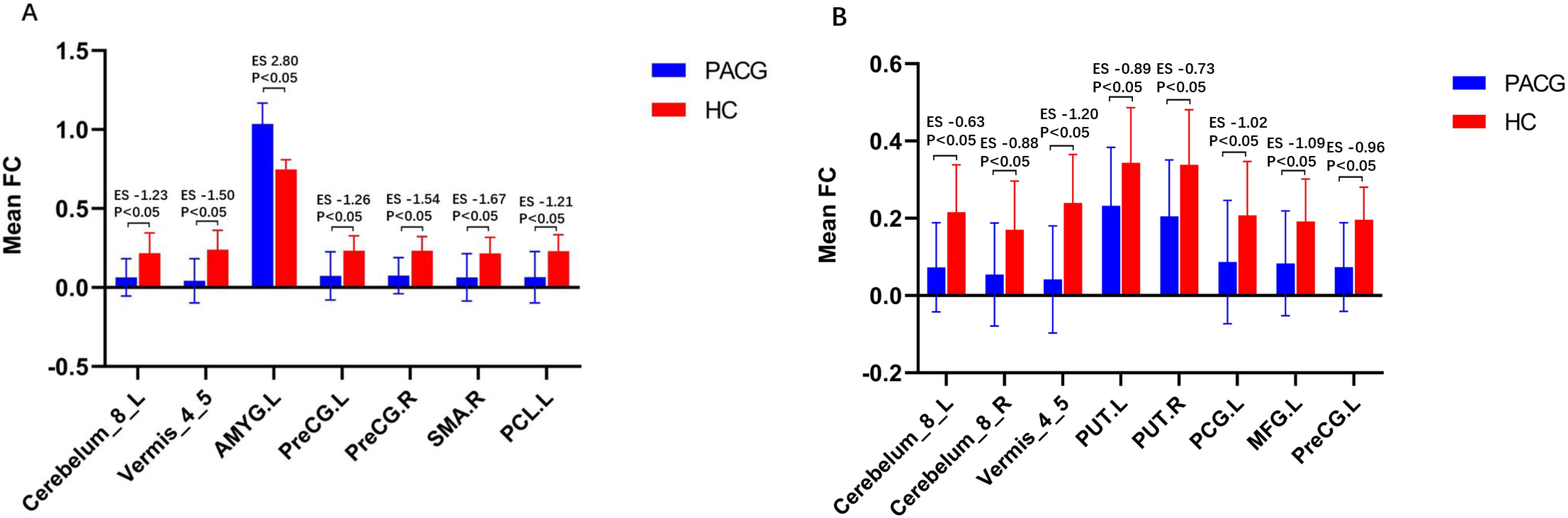
Mean weighted FC values for altered regions of the brain when comparing PACG patients and HC individuals using the right amygdala (A) or left amygdala (B) as the seed area. FC, functional connectivity; PACG, primary angle-closure glaucoma; HC, healthy control; AMYG, amygdala; PreCG, precentral gyrus; SMA, supplementary motor area; PCL, paracentral lobule; PUT, putamen; PCG, posterior cingulate gyrus; MFG, middle frontal gyrus; ES, effect size; L, left; R, right.
FC values for the left and right amygdalar seeds were not significantly correlated with disease duration, RNFLT, A-C/D, V-C/D, IOP, or any other evaluated clinical parameters.
Here, rs-fMRI approaches were used to evaluate alterations in whole brain and amygdalar FC in patients with PACG. This is the first systematic examination of such changes in the rs-FC of the amygdala in PACG patients to our knowledge. Overall, these analyses revealed extensive alterations in such amygdalar rs-FC in these individuals as compared to control subjects, consistent with the status of glaucoma as a degenerative disease that impacts higher-order cognitive brain functions rather than being restricted to those networks related to the visual field.
Relative to control subjects, patients with PACG exhibited reductions in FC between the right amygdala and bilateral PreCG, right SMA, and between the left amygdala and left PreCG. Both the PreCG and the SMA are components of the sensorimotor network (SMN). Prior reports have documented structural and functional associations between the amygdala and the PreCG [28, 29], which is a component of the amygdaloid-sensorimotor pathway that received amygdala-derived social-emotional stimulus-related inputs [30, 31]. A small number of studies have consistently found that the amygdala can facilitate and coordinate motor tasks via the SMA in the SMN of experimental animals [32, 33]. Glaucoma patients also reportedly exhibit dysregulated SMN function and structural characteristics. Huang et al. [34], for instance, determined that the ALFF area of the left precentral gyrus was reduced in PACG patients, with a corresponding increase in the PreCG ALFF area. Moreover, Dai et al. [35] determined that POAG patients exhibited reductions in FC between the primary visual cortex and the right PreCG, while Fu et al. [9] observed aberrant SMA ReHo values in individuals with PACG. The observed decrease in FC between the amygdala and these brain regions may thus be indicative of the PACG-related impairment of amygdala-sensorimotor pathways.
The left amygdala of individuals with PACG was also found to exhibit reduced FC with the left PCG and left MFG, which are both components of the default mode network (DMN) [36]. The DMN exhibits higher activity levels at rest, while it is less active when attention-demanding tasks are being performed [37]. It is also vital for emotional regulation, internal speech, the retrieval of episodic memories, planning for the future, and the contextualization of specific information [38]. Reductions in DMN activity have been linked to pain, sleep, cognitive impairment, and depression. In other studies, impaired FC between the amygdala and the DMN has been reported in hemodialysis patients with depression [39], childhood emotional abuse [40], major depressive disorder [41], and Alzheimer’s disease with comorbid depression [36, 42]. This suggests that DMN connectivity impairments are closely tied to the pathogenesis of depression. The present results align well with these past findings, suggesting that the reduced FC between the amygdala and the DMN may be reflective of the impairment of memory and emotional organization in PACG patients together with other potential self-cognitive processing defects. However, these explanations remain speculative at present, underscoring a need for future research more directly addressing the basis for these findings.
A reduction in FC was herein detected between the right amygdala and the left PCL in PACG patients. The PCL exhibits functional connections with other parietal and functional areas, and is responsible for controlling sensory and motor innervation while shaping spatial attention and motor functionality [43]. Decreased amygdalar FC with the PCL may be indicative of impaired attention and motor function in individuals diagnosed with PACG. In line with these findings, prior reports have indicated that PCL function may exhibit impairments in patients with glaucoma [44, 45, 46]. Although these data highlight the possibility that reductions in PCL FC values may be predictive of other forms of functional decline, additional research will be vital to clarify the ability of such reductions to predict PACG patient functional outcomes.
PACG patients enrolled in this study also exhibited reductions in rs-FC between the left amygdala and bilateral putamen. The putamen is a key component of the striatum, which comprises the reward network core and amplifies responses to negative emotions [47]. Much as is observed in Alzheimer’s disease and other neurodegenerative conditions [48], the putamen of patients with glaucoma exhibits altered structural and functional characteristics [49, 50]. Yang et al. [51] noted a reduction in putamen and amygdala volume in females with major depression together with a drop in amygdalar rs-FC with the putamen. Similarly, Dombrovski et al. [52] reported that major depressive disorder patients exhibiting suicidal ideation preented with a decrease in putamen bolume consistent with a potential link between depression-related loss of pleasure and reward network abnormalities. These results are consistent with the present findings, raising the possibility that the amygdala and striatal system in PACG patients present with functional and structural defects, ultimately compromising the amygdalostriatal circuits and thus potentially contributing to psychomotor dysfunction and anhedonia.
PACG patients also exhibited reductions in left amygdalar FC with the right cerebellum_8 and vermis_4_5, and in right amygdalar FC with the bilateral cerebellum_8 and vermis_4_5. Anatomical connections linking the amygdala and the cerebellar vermis have been documented previously [53]. The cerebellum is a central regulator of balance, motor control [54], and higher cognition [55]. The cerebellum is thus vital for effectively processing visual information [56, 57]. The observed PACG-related reductions in amygdalar FC with the cerebellum and vermis may thus be reflective of the impairment of cognitive function and visual attention in these patients. Chen et al. [58] previously documented a significant increase in DC values in the left cerebellum of individuals with PACG as compared to healthy subjects, while Fu et al. [9] noted significant cerebellar increases in ReHo values in patients with this form of glaucoma. Moreover, Dai et al. [35] observed a reduction in FC between the primary visual cortex and the left anterior cerebellum and verma in individuals diagnosed with POAG, while Song et al. [59] additionally detected a reduction in Right anterior cerebellar ReHo values and an increase in left posterior cerebellar ReHo values in POAG patients. The present data to not fully align with these prior results, potneitally suggesting that different subregions of the cerebellum play distinct roles in particular intrinsic connectivity networks [60].
Lastly, these results revealed the enhancement of FC between the left and right amygdala in individuals with PACG. High levels of interhemispheric amygdalar FC have previously been reported for weeks to months following pontine infarction in patients, raising the possibility that such abnormal connectivity may represent a neurological mechanism involved in cognitive compensation for motor impairment [61]. Localized neural plasticity may be similarly compensatory in the context of PACG. Such neuroplasticity refers to the selective ability of the nervous system to undergo structural and functional changes in response to injuries or experiences [62]. The decreased functionality of the amygdala and other regions of the brain in patients with PACG may thus trigger compensatory increases in bilateral amygdalar FC.
This study is subject to multiple limitations. For one, the degree of damage to retinal ganglion cells varies markedly among patients with glaucoma, and this damage can impair light perception, mood, and potentially amygdalar function in these patients. This variability may complicate efforts to clearly detect changes attributable to such retinal ganglion cell damage. In addition, light signals have been shown to impact amygdalar activity in a complex manner. In an effort to mitigate this effect, HCs and PACG patients in this study were exposed to similar lighting conditions. A mood scale was also not used to assess study participants, precluding any potential analysis of the relationship between changes in amygdalar FC and patient affective state. Lastly, this was a cross-sectional study such that the causal or temporal relationships between changes in brain activity and disease duration could not be established owing to the absence of appropriate neuroimaging follow-up data.
In conclusion, these results suggest that patients with PACG exhibit a reduction in FC between the amygdala and multiple regions of the grain including the cerebellum, SMA, putamen, MFG, PCG, anterior central gyrus, and paracentral lobule, whereas enhanced connectivity with the contralateral amygdala was detected in these same patients. As such, the pathophysiology of PACG may be associated with the disconnection and compensatory changes of higher-order regions of the amygdala. may be involved in the pathophysiological basis of PACG.
The raw data supporting the conclusions of this article will be made available by the authors, without undue reservation.
XZ and LC guided and designed the MRI experiment, besides, they reviewed and revised the manuscript. YC and YW analyzed the resting-state fMRI data. YC unscrambled the results and wrote the manuscript. LL, FO, ZX, and MY collected resting fMRI data and applied for the ethics. JL helped with statistics and graphing. All authors contributed to editorial changes in the manuscript. All authors read and approved the final manuscript. All authors have participated sufficiently in the work and agreed to be accountable for all aspects of the work.
The studies involving human participants were reviewed and approved by the Medical Research Ethics Committee of The First Affiliated Hospital of Nanchang University. The patients/participants provided their written informed consent to participate in this study (NO.2022 (3-008)).
The authors would like to thank all the patients and volunteers in the study for their helpful participation.
This study was supported by the Clinical Research Center for Medical Imaging in Jiangxi Province (No.20223BCG74001).
The authors declare no conflict of interest.
Publisher’s Note: IMR Press stays neutral with regard to jurisdictional claims in published maps and institutional affiliations.