- Academic Editor
†These authors contributed equally.
Background: To explore the value of a novel
ventricular-vascular coupling index (VVI) system in relation to age, gender and
body mass index (BMI). Methods: A total of 239 volunteers with
single-center and cross-sectional health screening were enrolled in the study.
Subjects were divided according to age (young [18–44 years], middle-age [45–59
years], old [60–80 years]), gender (male, female), and BMI (overweight/obese
[BMI
One of the most significant determinants of cardiovascular function is ventricular-vascular coupling. This term refers to the connection between the left ventricle and the arterial system, which is essential for measuring the energy efficiency of the left ventricle. The ventricular-vascular coupling of the heart maximized the energy efficiency [1]. Moreover, ventricular-vascular coupling is a potential therapeutic target for enhancing the performance of both the heart and vascular system and thus delaying the onset of heart failure [2]. The assessment of ventricular-vascular coupling includes several index components, such as effective arterial elastance (Ea), which indicates systemic arterial function, left ventricular end-systolic elastance (Ees), which reflects myocardial systolic function, and the ratio of Ea to Ees (Ea/Ees), which is known as the ventricular-vascular coupling index (VVI) [3].
Sunagawa et al. [4] first measured the “left ventricular end-systolic
pressure-volume” curve by using cardiac catheters in animal studies to calculate
Ea, Ees and VVI, thereby allowing evaluation of ventricular-vascular coupling.
Their findings, later corroborated by other researchers, showed that
ventricular-vascular decoupling was earlier than the onset of arterial or cardiac
pathogenesis [5]. However, the invasive nature of this method limited its
clinical application. It was suggested that the VVI obtained by deducing formula
based on echocardiography results had good concordance with the cardiac catheters
[6]. The mechanically explainable optimal cardiac work efficiency is often used
[7]. However, the VVI, defined as [(left ventricle end-systolic pressure (LVESP)/stroke volume (SV))/(LVESP/left ventricle end-systolic volum (LVESV)), LVESP = 0.9
In recent years, the arterial velocity pulse index (AVI) has emerged as a novel index of arterial stiffness. AVI has attracted considerable research attention as a possible alternative to PWV [14]. It is calculated non-invasively using cuff oscillometry, which quantitatively analyzes the oscillatory waves of proximal brachial artery cuff pressure to assess arterial elastance. AVI has been found to reflect central arterial pressure, and impaired AVI may indicate an increased cardiac load [15]. Earlier prospective studies showed that AVI is a powerful predictor of cardiovascular events in patients with hypertension combined with heart failure with preserved ejection fraction (HFpEF), and may improve their risk prediction [16]. Our previous research based on a large sample size found that AVI presents a “J” pattern with age, whereby it decreases before the age of 20 years and increases thereafter. This suggests a process of development and maturity of arterial elastance before the age of 20 [17]. Furthermore, AVI has been found to reflect gender- and age-related differences in arterial elastance, and to independently predict the risk of cardiovascular events over the following 10 years [18]. AVI also has the advantages of simple operation, low operator dependence, and good reproducibility of measurement, thus making it a promising tool for large-scale population monitoring or screening [19].
In this study, we hypothesized that a novel cardiovascular coupling index system consisting of AVI, LVGLS and AVI/LVGLS provides a more sensitive reflection of the impact of age on cardiovascular function compared to traditional, echocardiography-derived Ea, Ees and VVI. Age, gender and BMI are known to influence both arterial and cardiac function. Hence, the aim of this study was therefore to investigate the effect of age, gender and BMI on ventricular-vascular coupling using the new index system in a healthy volunteer population. The findings of this study should provide novel perspectives for the systematic evaluation of cardiac performance.
A single-center, cross-sectional study design was used. Healthy volunteers from
the medical examination center of Jiading Branch of Shanghai General Hospital
between January 2022 and January 2023 were selected as the study subjects.
Demographic information including age, gender, height and weight was recorded for
each participant using electronic data capture technology. BMI for each
participant was calculated as weight (kg)/square height (m
A commercially available system (EPIQ7, Philips, S5 probe, Amsterdam, Netherlands)
with a frequency of 1–5 MHz, a frame frequency of
Mean arterial pressure (MAP) was calculated as [(2
Transthoracic acquisitions of three consecutive long-axis images (apical four-chamber, three-chamber, and two-chamber) were performed during image capture, with careful attention paid to the absence of arrhythmia during image capture. Quantitative analysis software was used for off-line analysis. Specifically, the endocardial border of the apical four-chamber, two-chamber, and three-chamber views was manually traced and adjusted at end-systole. Longitudinal strain curves were automatically processed and the LVGLS value from all three views was calculated. Negative strain values indicate left ventricular myofiber shortening. To ensure the accuracy and reliability of the measurements, LVGLS was measured independently by two experienced doctors (WL with 10 years of experience, and XL with 12 years of experience) in a blinded fashion and without knowledge of the subjects’ information.
The AVI was obtained using PASESA AVE-2000Pro (Shisei Datum, Tokyo, Japan). Participants were seated and allowed to rest for 5 minutes before placing their left upper arm on the cuff. Their name, age, gender, height, and weight information were inputted. The instrument automatically obtained the AVI after pressing the “measure” button. Each subject’s measurements were repeated at 5-minutes intervals, and the average of three measurements was calculated (Fig. 1).
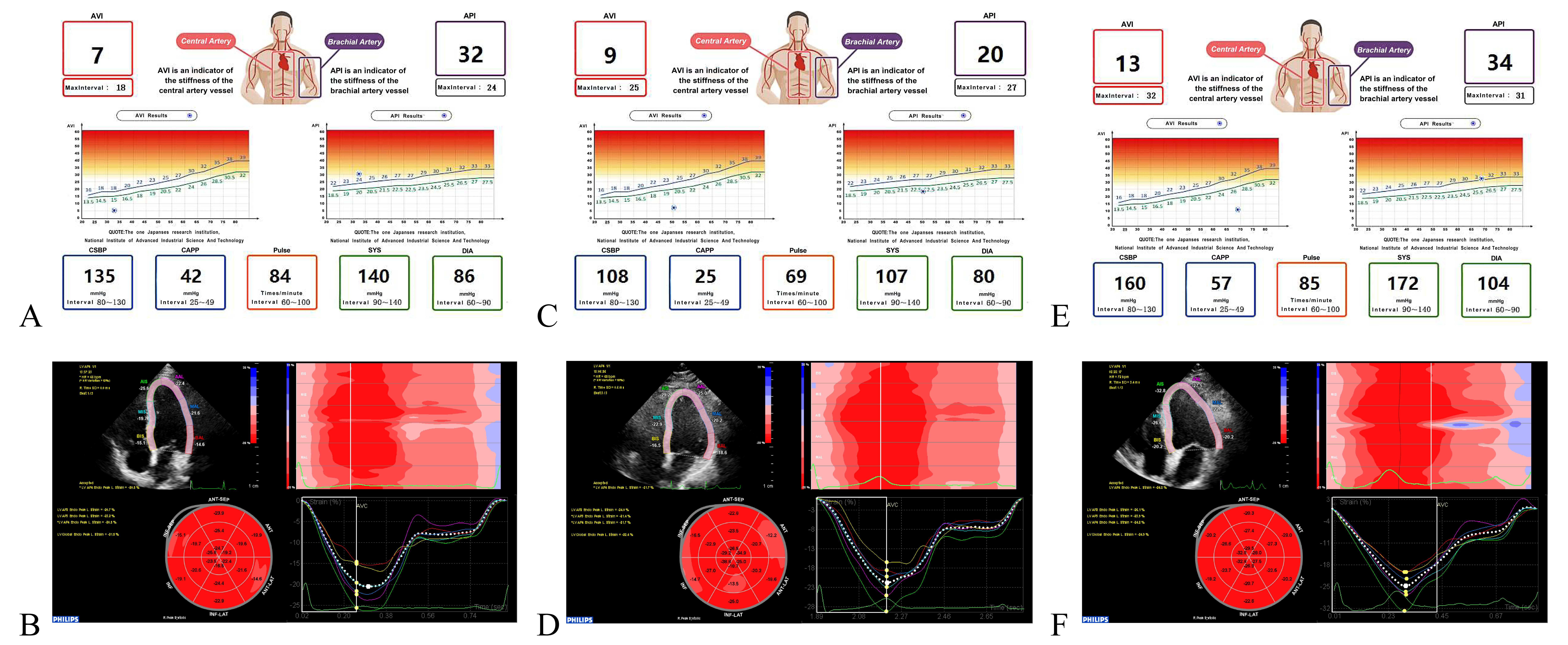
Analysis of AVI and LVGLS. (A,B) Male, 39 years, AVI = 7, LVGLS = –21.5%, AVI/LVGLS = –32.56. (C,D) Female, 48 years, AVI = 9, LVGLS = –22.4%, AVI/LVGLS = –40.18. (E,F) Female, 65 years, AVI = 13, LVGLS = –24.9%, AVI/LVGLS = –34–52.21. AVI, arterial velocity pulse index; LVGLS, left ventricular global longitudinal strain; API, arterial pressure volume index; CSBP, central systolic blood pressure; CAPP, central artery pulse pressure; SYS, systolic; DIA, diastolic.
The proposed VVI index was calculated as the ratio of the arterial stiffness measured by AVI, and the myocardial performance estimated with LVGLS (AVI/LVGLS ratio). This was compared with the widely used VVI calculated by echocardiography as described above.
Twenty subjects were randomly selected and AVI and LVGLS were measured by two physicians for inter-group repeatability. One week later, AVI and LVGLS measurements were repeated by one of the physicians on the same 20 subjects to assess intra-group repeatability.
SPSS 23.0 (IBM, Armonk, NY, USA) statistical software was used for statistical
analyses. Normality and homogeneity of variance were tested for quantitative
data, expressed as the mean and standard deviation. Comparison of the means
between two groups was performed using t-test, while comparison of the
means among three groups was performed using one-way analysis of variance.
Pairwise comparison of means between groups was conducted using LSD-q
test. Qualitative data were represented as examples, and the chi-square test was
used for comparison. Pearson correlation analysis was used for univariate
correlation analysis, and multiple stepwise linear regression analysis was used
for multivariate correlation analysis. Bland-Altman and linear regression
analysis were used to assess the repeatability of AVI and LVGLS measurements. The
diagnostic efficacy of AVI and Ea, LVGLS and Ees, AVI/LVGLS and VVI for
differentiating changes in cardiovascular function in subjects from different age
groups was evaluated by ROC curve. A two-sided p-value of
A total of 239 subjects were included in this study, consisting of 109 males
(45.6%) and 130 females (54.4%) with a mean age of 46.16
The general factors, cardiac structure and functional indicators of subjects from the different age groups are summarized in Table 1. The old group had 52 subjects, of which 48 (96.2%) were aged 60–69 years and 4 (3.8%) were aged 70–80 years. Compared to the young group, the middle-age and old groups had significantly higher Ea values, indicating increased arterial stiffness. No significant differences in Ees and VVI were observed between the different age groups. AVI increased progressively with age and the highest value was observed in the elderly group, suggesting that arterial stiffness was greatest in this group. LVGLS was lower in both the middle-age and old groups compared with the young group, indicating impaired myocardial function. The AVI/LVGLS ratio was smallest in the young group, followed by the middle-aged and then the old group. The difference in AVI/LVGLS ratio between the three groups was statistically significant, suggesting it can identify and reflect the ventricular-vascular coupling relationship in subjects with different ages (Table 1).
Items | Young group (n = 112) | Middle-age group (n = 75) | Old group (n = 52) | F/ |
p-value | |
Female (n, %) | 56 (50%) | 46 (61%) | 28 (54%) | 2.155 | 0.340 | |
Height (cm) | 167.15 |
161.83 |
163.60 |
10.514 | ||
Weight (kg) | 64.60 |
62.08 |
60.81 |
2.432 | 0.092 | |
BMI (kg/m |
22.99 |
23.61 |
22.66 |
1.544 | 0.216 | |
BSA (m |
1.69 |
1.63 |
1.62 |
3.937 | 0.022 | |
Heart rate (beats/min) | 75.07 |
69.60 |
70.59 |
4.909 | 0.008 | |
SBP (mmHg) | 130.03 |
132.45 |
133.73 |
0.712 | 0.492 | |
DBP (mmHg) | 85.42 |
86.83 |
79.94 |
4.839 | 0.009 | |
MAP (mmHg) | 100.29 |
102.04 |
97.87 |
1.396 | 0.250 | |
Echocardiography | ||||||
LVEDD (mm) | 45.44 |
45.60 |
45.17 |
0.218 | 0.804 | |
LVESD (mm) | 28.40 |
28.34 |
28.42 |
0.013 | 0.987 | |
LVEDV (mL) | 75.98 |
69.76 |
66.33 |
5.349 | 0.006 | |
LVESV (mL) | 28.31 |
26.83 |
26.48 |
0.607 | 0.546 | |
SV (mL) | 47.67 |
42.93 |
39.86 |
7.911 | 0.001 | |
LVM (g) | 134.46 |
135.82 |
136.66 |
0.113 | 0.893 | |
E/A ratio | 1.58 |
1.12 |
0.96 |
41.953 | ||
TDI e |
15.26 |
11.66 |
10.00 |
94.573 | ||
LVEF (%) | 0.63 |
0.62 |
0.60 |
1.564 | 0.212 | |
Ventricular-vascular coupling | ||||||
Ea (mmHg/mL) | 2.64 |
3.05 |
3.13 |
6.756 | 0.002 | |
Ees (mmHg/mL) | 4.54 |
5.37 |
4.99 |
2.900 | 0.060 | |
VVI | 0.62 |
0.63 |
0.68 |
1.348 | 0.262 | |
LVGLS (%) | –22.79 |
–21.45 |
–20.57 |
5.189 | 0.006 | |
AVI | 10.11 |
13.69 |
15.98 |
37.098 | ||
AVI/LVGLS ratio | –46.76 |
–65.84 |
–80.19 |
27.851 |
Note: Young group subjects were aged 18 to 44 years; Middle-age group subjects
were aged 45 to 59 years; Old group subjects were aged 60 to 80 years.
Echocardiography, the index of left ventricular structure and
function were obtained by Echocardiography;
Ventricular-vascular coupling, the traditional and novel index
system of ventricular-vascular coupling; BMI, body mass index; BSA, body surface
area; SBP, systolic blood pressure; DBP, diastolic blood pressure; MAP, mean
arterial pressure; LVEDD, left ventricle end-diastolic dimension; LVESD, left
ventricle end-systolic dimension; LVEDV, left ventricle end-diastolic volume;
LVESV, left ventricle end-systolic volume; SV, stroke volume; LVM, left
ventricular mass; E/A, E and A mitral inflow waves by
Doppler; TDI, Tissue Doppler Imaging; e
We next investigated possible gender differences in AVI/LVGLS. Males showed significantly greater values for the general factors (height, weight, BMI, SBP, DBP, MAP) and left ventricular structural indices (LVEDD, LVESD, LVEDV, LVESV, SV and LVM) compared to the female subjects (Table 2). Both Ea and Ees were greater in female subjects than in male subjects. No significant gender difference was observed for VVI. Females showed higher LVGLS than males, suggesting their myocardial function was stronger than in men. No significant differences were observed between males and females for AVI and AVI/LVGLS (Table 2).
Items | Females (n = 131) | Males (n = 108) | t value | p-value | |
Age (years) | 46.56 |
45.40 |
0.658 | 0.511 | |
Height (cm) | 159.60 |
170.83 |
–14.031 | ||
Weight (kg) | 56.79 |
70.33 |
–10.863 | ||
BMI (kg/m |
22.31 |
24.07 |
19.817 | ||
BSA (m |
1.55 |
1.79 |
–12.889 | ||
Heart rate (beats/min) | 72.27 |
72.88 |
–0.378 | 0.706 | |
SBP (mmHg) | 129.04 |
134.49 |
–2.126 | 0.035 | |
DBP (mmHg) | 81.71 |
88.09 |
–3.895 | ||
MAP (mmHg) | 97.48 |
103.56 |
–3.444 | 0.001 | |
Echocardiography | |||||
LVEDD (mm) | 43.96 |
47.20 |
–7.610 | ||
LVESD (mm) | 26.97 |
30.09 |
–9.117 | ||
LVEDV (mL) | 65.47 |
78.43 |
–4.667 | ||
LVESV (mL) | 24.43 |
30.68 |
–4.475 | ||
SV (mL) | 41.05 |
47.75 |
–3.683 | ||
LVM (g) | 122.48 |
150.77 |
–8.336 | ||
E/A ratio | 1.33 |
1.26 |
1.063 | 0.289 | |
TDI e |
13.12 |
12.97 |
0.314 | 0.753 | |
LVEF (%) | 0.63 |
0.61 |
1.718 | 0.087 | |
Ventricular-vascular coupling | |||||
Ea (mmHg/mL) | 3.05 |
2.73 |
2.329 | 0.021 | |
Ees (mmHg/mL) | 5.42 |
4.40 |
3.519 | 0.001 | |
VVI | 0.61 |
0.66 |
–1.634 | 0.104 | |
LVGLS (%) | –22.58 |
–20.89 |
–3.060 | 0.003 | |
AVI | 12.35 |
12.73 |
–0.589 | 0.556 | |
AVI/LVGLS ratio | –57.49 |
–65.33 |
1.825 | 0.070 |
Note: Echocardiography, the index of left ventricular structure
and function were obtained by Echocardiography;
Ventricular-vascular coupling, the traditional and novel index
system of ventricular-vascular coupling; BMI, body mass index; BSA, body surface
area; SBP, systolic blood pressure; DBP, diastolic blood pressure; MAP, mean
arterial pressure; LVEDD, left ventricle end-diastolic dimension; LVESD, left
ventricle end-systolic dimension; LVEDV, left ventricle end-diastolic volume;
LVESV, left ventricle end-systolic volume; SV, stroke volume; LVM, left
ventricular mass; E/A, E and A mitral inflow waves by
Doppler; TDI, Tissue Doppler Imaging; e
To investigate the impact of overweight/obesity on ventricular-vascular
coupling, we divided subjects into two groups according to a BMI threshold of 24,
namely an overweight/obese group (BMI
Items | Control group | Overweight/Obese group | t value | p-value | |
(n = 157) | (n = 82) | ||||
Age (years) | 46.13 |
45.85 |
0.151 | 0.880 | |
Height (cm) | 163.80 |
166.34 |
–2.259 | 0.025 | |
Weight (kg) | 57.24 |
73.77 |
–13.328 | ||
BSA (m |
1.58 |
1.81 |
–11.018 | ||
Heart rate (beats/min) | 73.38 |
70.99 |
1.375 | 0.170 | |
SBP (mmHg) | 129.81 |
134.83 |
–1.860 | 0.064 | |
DBP (mmHg) | 83.12 |
87.49 |
–2.493 | 0.013 | |
MAP (mmHg) | 98.68 |
103.27 |
–2.447 | 0.015 | |
Echocardiography | |||||
LVEDD (mm) | 44.52 |
47.16 |
–5.640 | ||
LVESD (mm) | 27.68 |
29.72 |
–5.296 | ||
LVEDV (mL) | 68.71 |
76.76 |
–2.662 | 0.008 | |
LVESV (mL) | 26.09 |
29.68 |
–2.356 | 0.019 | |
SV (mL) | 42.62 |
47.08 |
–2.131 | 0.035 | |
LVM (g) | 127.01 |
151.07 |
–6.574 | ||
E/A ratio | 1.32 |
1.26 |
0.785 | 0.433 | |
TDI e |
13.24 |
12.70 |
1.142 | 0.255 | |
LVEF (%) | 0.62 |
0.61 |
0.734 | 0.464 | |
Ventricular-vascular coupling | |||||
Ea (mmHg/mL) | 2.94 |
2.83 |
0.727 | 0.468 | |
Ees (mmHg/mL) | 5.12 |
4.60 |
1.668 | 0.097 | |
VVI | 0.63 |
0.65 |
–0.747 | 0.456 | |
LVGLS (%) | –22.13 |
–21.16 |
–1.651 | 0.100 | |
AVI | 12.12 |
13.30 |
–1.731 | 0.085 | |
AVI/LVGLS ratio | –58.60 |
–66.12 |
1.660 | 0.099 |
Note: Control group were subjects with BMI
As shown in Fig. 2A, a significant inverse relationship was observed between
AVI/LVGLS and SBP in young subjects (r = –0.399, p
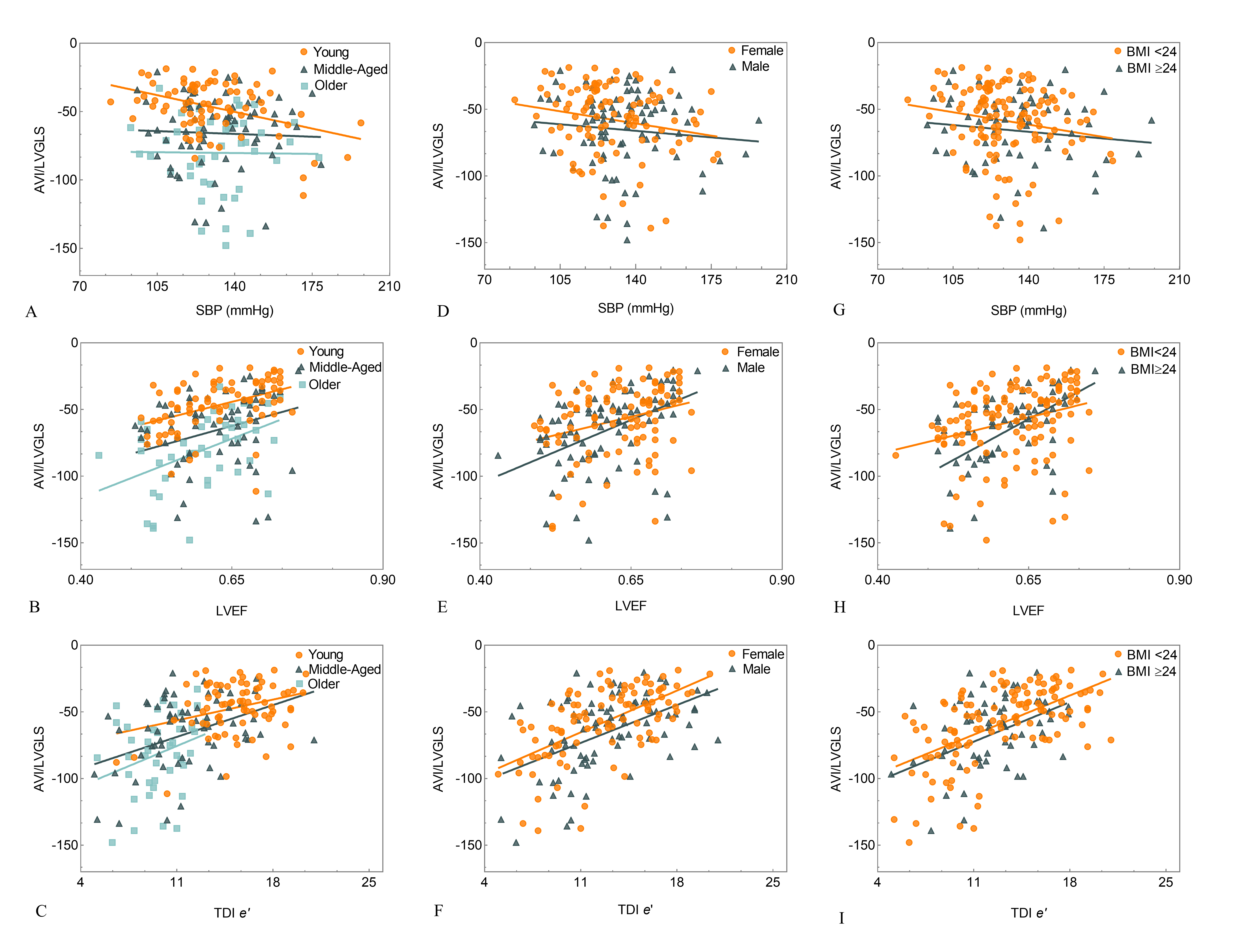
Correlation between the AVI/LVGLS ratio and SBP, LVEF,
TDI e
No significant correlation was found between AVI/LVGLS and SBP in either males or
females (r = –0.086 and –0.193, respectively; p
AVI/LVGLS was not significantly correlated with SBP in either the control or
overweight/obese groups (r = –0.171 and –0.103, respectively; p
In all subjects, AVI/LVGLS was negatively correlated with age, SBP, LVEDV, LVESV,
Ea and VVI, (r = –0.454, –0.153, –0.149, –0.323, –0.132 and –0.371,
respectively; all p
Variables | Univariable | Multivariable | ||||
r | p-value | Regression coefficient ( |
Standardized coefficients ( |
95% Confidential interval | p-value | |
Age | –0.454 | –0.482 | –0.215 | (–0.817–0.147) | 0.005 | |
BMI | –0.120 | 0.102 | –0.035 | - | - | 0.537 |
Heart rate | 0.007 | 0.921 | –0.036 | - | - | 0.539 |
SBP | –0.153 | 0.037 | –0.016 | - | - | 0.795 |
MAP | –0.062 | 0.403 | –0.003 | - | - | 0.963 |
LVEF | 0.367 | –0.068 | - | - | 0.491 | |
E/A | 0.361 | 0.010 | - | - | 0.888 | |
LVEDV | –0.149 | 0.042 | –0.105 | - | - | 0.512 |
LVESV | –0.323 | –1.492 | –0.509 | (–1.857 – –1.127) | ||
TDI e |
0.504 | 2.953 | 0.350 | (1.709–4.198) | ||
Ea | –0.132 | 0.072 | –8.365 | –0.261 | (–12.466 – –4.265) | |
Ees | 0.146 | 0.046 | –0.088 | - | - | 0.405 |
VVI | –0.371 | 0.097 | - | - | 0.338 |
Note: BMI, body mass index; SBP, systolic blood pressure; MAP, mean arterial
pressure; LVEF, left ventricular ejection fraction; E/A, E and A
mitral inflow waves by Doppler; LVEDV, left ventricle end-diastolic volume; LVESV,
left ventricle end-systolic volume; TDI, Tissue Doppler Imaging; e
AVI/LVGLS had significantly superior diagnostic performance compared to VVI in
young and middle-age subjects, with areas under the ROC curve of 0.709 and 0.519,
respectively (p
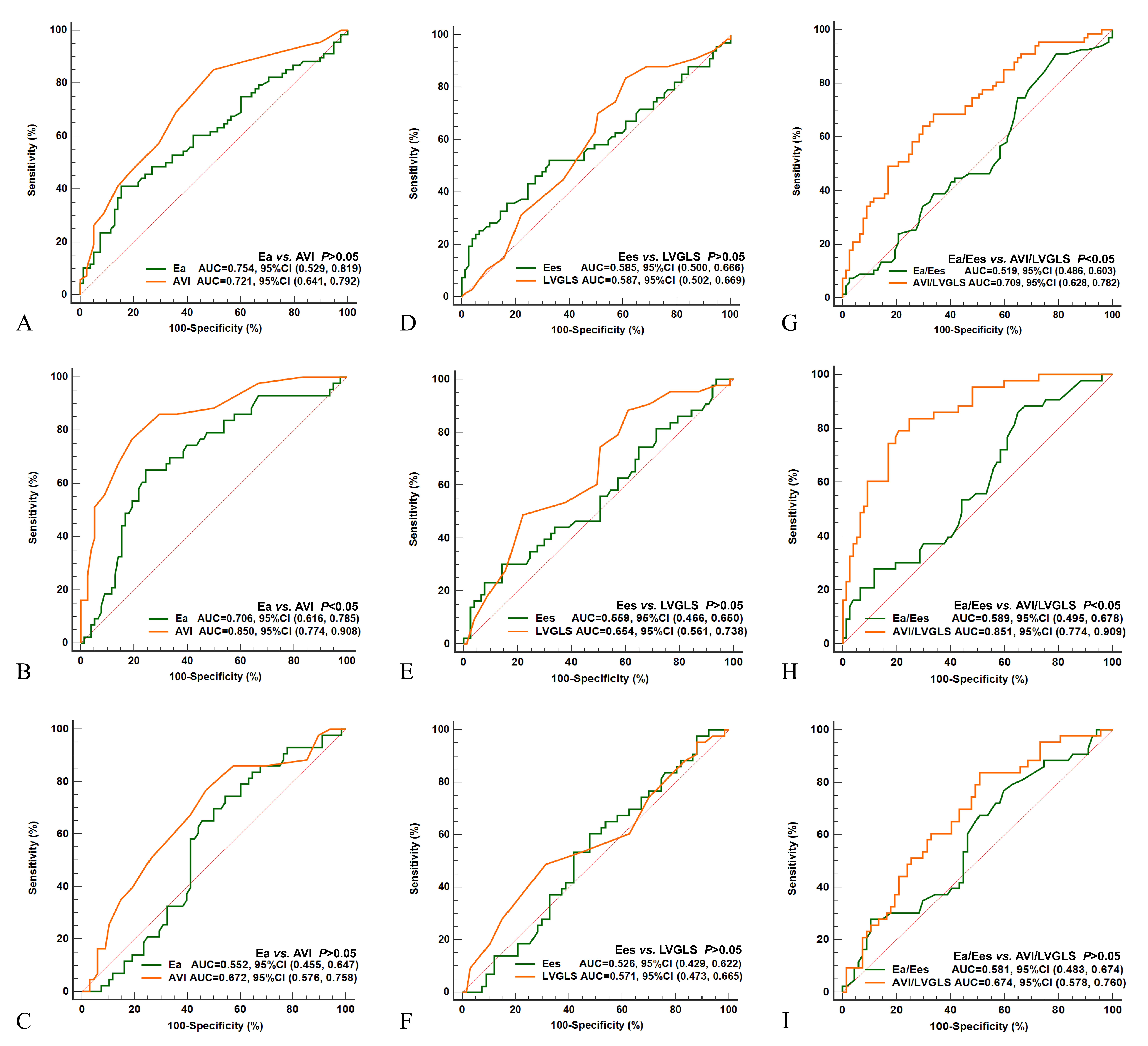
ROC curves for the parameters of arterial and ventricular coupling. ROC curves for Ea and AVI in young and middle-age groups (A), young and old groups (B), and middle-age and old groups (C). ROC curves for Ees and LVGLS in young and middle-age groups (D), young and old groups (E), and middle-age and old groups (F). ROC curves for VVI and AVI/LVGLS in young and middle-age groups (G), young and old groups (H), and middle-age and old groups (I). Ea, effective arterial elastance; Ees, left ventricular end-systolic elastance; AVI, arterial velocity pulse index; LVGLS, left ventricular global longitudinal strain; VVI, ventricular-vascular coupling index; ROC, receiver operating characteristic curve; AUC, area under curve.
In the young and elderly subjects, the diagnostic efficacy of AVI/LVGLS was higher than that of VVI (Fig. 3H), while that of AVI was higher than Ea (Fig. 3B). There was no significant difference in diagnostic efficacy between LVGLS and Ees (Fig. 3E).
In middle-age and old subjects, no significant differences in diagnostic
efficacy were found between AVI/LVGLS and VVI, AVI and Ea, and LVGLS and Ees (all
p
The repeatability of AVI was evaluated by inter- and intra-group comparisons.
These revealed a high consistency in the measurements [between: R
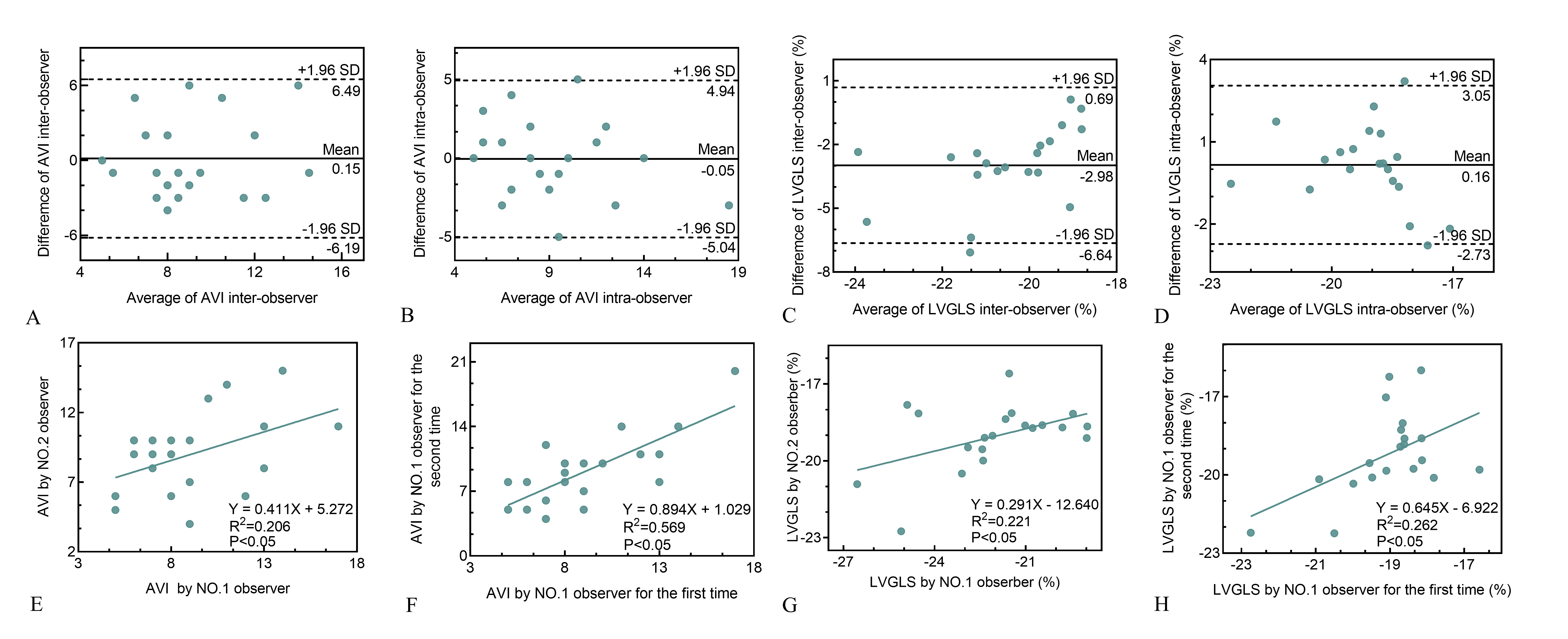
Repeatability test of AVI and LVGLS by Bland-Altman plot and linear correlation analysis. Bland-Altman analysis (A,B) showed a consistent trend and linear regression analysis (E,F) showed good agreement for AVI inter-observer and intra-observer respectively. Bland-Altman analysis (C,D) showed a consistent trend and linear regression analysis (G,H) showed good agreement for LVGLS inter-observer and intra-observer respectively. AVI, arterial velocity pulse index; LVGLS, left ventricular global longitudinal strain.
Similarly, the repeatability of LVGLS was assessed by between- and within-group
comparisons. These revealed a high consistency in the measurements [between:
R
The main findings of this study were: (1) AVI, LVGLS, and AVI/LVGLS showed
age-related changes, with AVI and AVI/LVGLS increasing with age and LVGLS decreasing.
Our study suggests that arterial stiffness increases with age, while left
ventricular systolic function decreases and the ratio of the two increases,
thereby reflecting age-related changes in the cardiovascular system. AVI/LVGLS was
found to increase with age and correlated inversely and independently with age.
LVESV and Ea were positively correlated with TDI e
AVI provides a more direct assessment than Ea of the total elastance of the arterial tree and the resistance of peripheral arteries, both of which are closely related to cardiac function. The present study revealed that the novel arterial stiffness index AVI was more sensitive than Ea in identifying the degree of arterial aging in subjects with different ages. Furthermore, it was more stable in its assessment of differences in arterial elastance between genders. Arterial stiffness increases with age [9] and is also promoted by various factors associated with aging, such as increased arterial wall stress, oxidative stress, and inflammatory stimuli. These factors decrease the elastic fibers in arteries that maintain vascular compliance, whereas the collagen fibers increase. This leads to changes in the structure and function of the arterial wall, resulting in increased vascular stiffness and decreased compliance [25, 26]. AVI, a novel index of arterial stiffness, was found in this study not only to reflect the stiffness of the central artery, but also to correlate significantly with total peripheral vascular impedance and left ventricular contractility [15]. Previous studies have shown that AVI is a sensitive predictor of the risk of subclinical coronary atherosclerosis without significant obstruction in the lumen [22]. Furthermore, AVI is independently associated with the concentration of plasma atrial natriuretic peptide and with a history of congestive heart failure [27], suggesting that enhanced AVI may reflect an increase in cardiac workload. The present study also demonstrated that Ea could differentiate vascular elastance between young and middle-age individuals, but not between middle-age and old individuals. This result suggests that Ea has limitations for evaluating the elastance of arteries in middle-age and elderly people. Ea reflects the elastance of the arterial tree and the resistance of peripheral vessels, as well as reflecting the overall afterload of the heart [4]. It is determined by the formula Ea = LVESP/SV, which reflects only the reserve function of the heart SV [24]. Ea may have reduced diagnostic efficacy in middle-age and elderly individuals due to a decrease in cardiac stroke reserve [9]. Additionally, the inadequate assessment of systemic common artery elastance by Ea limits its clinical application [24].
LVGLS has greater sensitivity than Ees for the evaluation of impaired cardiac systolic function [5]. Of note, LVGLS has the ability to differentiate cardiac function across different age groups, as well as between genders. Various studies have confirmed that LVGLS enables earlier detection of impaired systolic function and can thus function as an early warning for cardiovascular and cerebrovascular events [28, 29]. LVGLS, as a contrasting predictor of cardiovascular events, has been incorporated into guidelines for the early assessment of cardiac ventricular disorders [30]. Reduced LVGLS serves as a partial manifestation of impaired systolic function in patients with HFpEF [31]. Moreover, a follow-up study of 4172 patients with acute heart failure revealed that LVGLS alone was an independent predictor of all-cause mortality at 5 years, regardless of whether or not LVEF was normal [32]. Myocardial compliance decreases as cardiomyocytes undergo apoptosis or necrosis with aging and as the extracellular matrix undergoes fibrotic remodeling, thus leading to functional changes [33]. The present study found that Ees was higher in middle-age and old subjects compared to young subjects. Ees reflects ventricular contractility and systolic stiffness, and is an essential parameter for assessing cardiac systolic function and hemodynamic status. However, Ees does not take into account myocardial geometry and tissue structure (such as cardiomyocytes and elastic fibers), both of which have a significant impact on ventricular systolic and diastolic function [6]. The present study found that ventricular end-diastolic volume decreased with increasing age, while the ejection fraction remained unchanged. This finding explains why Ees behaves differently across different age groups and in obese individuals.
An increased AVI is indicative of increased arterial stiffness, while decreased
absolute LVGLS values suggest impaired ventricular myocardial systolic function and
are also associated with diastolic dysfunction [31]. The present study examined
the interaction between AVI and LVGLS, which represents ventricular-vascular
interactions. Possible reciprocal mechanisms include increased arterial
stiffness, decreased peripheral vascular compliance, increased ventricular
afterload, decreased coronary perfusion and myocardial oxygen delivery. These
result in cardiomyocyte hypertrophy, fibroblast growth and interstitial fibrosis,
leading to ventricular hypertrophy, and systolic and diastolic dysfunction [7].
Based on this, the AVI/LVGLS ratio is closely associated with left ventricular
diastolic function indicators such as E/A and TDI e
It is worth noting that the AVI/LVGLS ratio, a novel index system for
ventricular-vascular coupling, is consistent with previous VVI obtained through
echocardiography. Moreover, in healthy populations it does not differ
significantly according to gender or BMI. There are several potential
explanations for this observation. Firstly, overweight/obese individuals and
those with metabolic diseases may have impaired subclinical myocardial
contractility which can affect LVGLS [30]. Studies have shown that bariatric
surgery can improve LVGLS in severely obese individuals (BMI
There are several limitations to this study. First, this was an exploratory study aimed at establishing the basic clinical value of the cardiac-vascular coupling index system. It was therefore a preliminary study conducted on the normal population, and its application in various diseases has yet to be investigated. We plan to conduct further research on the characteristics of these indexes in patient cohorts with common diseases such as diabetes and hypertension. Second, the VVI measurement in this study was obtained by echocardiography rather than by using the gold standard invasive pressure-volume curve loop. Our novel index system for ventricular-vascular coupling is non-invasive, simple, and highly applicable for extensive clinical work and large-scale community screening. Third, our novel index system was not compared with previous PWV/LVGLS, which we plan to investigate in future studies.
The novel cardiac-vascular coupling index system described in this study is more effective at identifying age-related changes in cardiovascular disease compared to the traditional system. Moreover, it is not affected by gender or overweight/obesity. Among healthy individuals, the AVI/LVGLS index is a more sensitive and reliable diagnostic tool than VVI for assessing cardiac-vascular couplings at different ages, and is independently associated with left ventricular diastolic dysfunction. The use of AVI, LVGLS and AVI/LVGLS indexes may provide a straightforward and reproducible way to evaluate cardiovascular function status. Moreover, these indexes could offer new perspectives for investigating cardiovascular reserve function in patients with various diseases.
All data generated or used during the study appear in the submitted article.
ZJL and XHL conceived of and designed the study; LHW drafted the manuscript; MJZ and JXC performed statistical design and analysis. LHW, JXC, LJ, CQS and JLS coordinated study staff and data curation. MJZ and LJ are performed the research. LFD participated in data, image quality supervision. All authors contributed to editorial changes in the manuscript. All authors read and approved the final manuscript. All authors have participated sufficiently in the work to take public responsibility for appropriate portions of the content and agreed to be accountable for all aspects of the work in ensuring that questions related to its accuracy or integrity.
The study protocol was approved by the Ethics Committee of Shanghai General Hospital (2021KY057). All patients gave written informed consent.
Not applicable.
This work was supported by Natural Science Foundation of Shanghai (21ZR1451400), Shanghai Health and Family Planning Commission Fund (202240235), and Shanghai Jiading District Health and Family Planning Commission Fund (2021-KY-10).
The authors declare no conflict of interest.
Publisher’s Note: IMR Press stays neutral with regard to jurisdictional claims in published maps and institutional affiliations.