- Academic Editor
†These authors contributed equally.
Background: Mitral regurgitation (MR) has a high prevalence and
aggravates hypoperfusion and hypoxia in heart failure (HF). Renal tubular
epithelial cells are sensitive to hypoxia, and therefore tubulointerstitial
damage is quite common in HF. However, the correlation between tubular
dysfunction and MR has not been studied. The aim of this work was to evaluate the
prognostic significance of urinary N-acetyl-
Despite major advances in pharmacotherapies and device treatments, the prognosis for heart failure (HF) remains poor. Persistent left ventricular remodeling and mitral annular dilation cause mitral regurgitation (MR). Secondary/functional mitral regurgitation (FMR) reportedly has a prevalence ranging from 17% to 53% in both acute and chronic HF [1, 2, 3], leading to reduced quality of life, a high mortality rate, and dismal prognosis [4]. Previous studies have suggested that MR may be an indicator of the severity of potential ventricular disease, as well as exerting an effect on disease progression [2].
MR increases the left ventricular preload and decreases the forward flow, resulting in hypofusion and hypoxic damage to renal parenchyma and interstitium. It causes elevated pressures in the left atrial (LA), as well as pulmonary vascular resistance and right-sided heart. These effects transmit to the kidney and lead to increased renal venous and interstitial pressures, thereby contributing to “congestive renal failure” [5]. Activation of the sympathetic nervous system and the renin-angiotensin-aldosterone system due to circulation congestion and volume overload also causes undesirable effects to the kidney. Earlier reports have linked endothelial dysfunction to MR [6] and its potential effects on end organs like the kidney [7].
Several investigators have identified important biomarkers and prognostic
factors for HF and MR, including natriuretic peptides, troponin T, the New York
Heart Association functional class, anemia, left ventricular ejection fraction
N-acetyl-
This was an observational prospective study with consecutive enrollment at a single-center. Adult HF patients admitted to the Department of Cardiology, the Second Affiliated Hospital, Zhejiang University School of Medicine between July 31, 2019 and November 11, 2021 were included in the study. All patients in which echocardiography suggested the presence of MR were included (n = 461). Patients who withdrew their informed consent (n = 26) or for whom the uNAG measurement was not available (n = 45) were excluded, leaving 390 participants. The study conformed to the Declaration of Helsinki and was approved by the Institutional Review Board of the Second Affiliated Hospital of Zhejiang University. Written informed consent was provided by all patients.
In accordance with the ESC [22] and Chinese guidelines [23], a diagnosis of HF
was based on the description of symptoms (chest tightness, dyspnea, exercise
intolerance), physical examination (pulmonary rales or peripheral edema),
laboratory measurements (B-type natriuretic peptide (BNP)
MR was assessed quantitatively using the proximal isovelocity surface area
(PISA) to calculate mitral regurgitation volume (RVol) and the effective
regurgitation orifice area (EROA). The severity of MR was classified as grade 0
for no regurgitation, grade 1 for mild regurgitation (EROA
Baseline clinical data included the patient characteristics of age, gender,
body-mass index (BMI), systolic blood pressure (SBP), diastolic blood pressure
(DBP), heart rate, New York Heart Association (NYHA) functional class,
comorbidities (diabetes, hypertension, atrial fibrillation, coronary artery
disease (CAD) and chronic kidney disease (CKD, adjudicated according to medical
records or estimated glomerular filtration rate (eGFR)
Venous blood and spot urine samples were obtained in the morning within 24 h of admission and immediately sent to the hospital’s central laboratory for measurement of routine clinical parameters. These included hemoglobin (Hb), NT-proBNP, C-reactive protein (CRP), serum sodium, serum creatinine (Scr), blood urea nitrogen (BUN), urinary NAG and microalbumin. Urinary NAG was measured with the NAG kit (MPT) as per the manufacturer’s instructions (Beijing Leadman Biochemistry Technology Co. Ltd. Beijing, China) on a Beckman Coulter instrument AU5800 (Beckman Coulter, Brea, CA, USA). Urinary microalbumin was measured using scatter turbidimetry on a special protein analyzer (BNII SYSTEM, Siemens, Munich, Germany). The Chronic Kidney Disease Epidemiology Collaboration (CKD-EPI) formula was used to calculate eGFR [25].
A standard echocardiogram (Philips IE-33 color Doppler ultrasound imaging instrument, equipped with X-1 probe, S5 probe) was performed prior to discharge. In addition to the left ventricular ejection fraction (LVEF, based on the modified Simpson method), echocardiogram parameters included left atrium dimension (LAD), left ventricular end-diastolic volume (LVEDV), left ventricular internal diameter in diastolic phase (LVIDd), and left ventricular internal diameter in systolic phase (LVIDs). Echocardiography was performed and confirmed by experienced cardiac sonographers, with any discordant cases consulted further by a third sonographer.
The primary endpoint was the composite of all-cause death (defined as death from any cause) or HF rehospitalization (defined as an inpatient admission with exacerbation of HF symptoms and requirement for treatment with intravenous diuretics or inotropic agent), while secondary outcomes included all-cause death and HF rehospitalization. All patients were followed up by outpatient visits or telephone contact at 1, 3, and 6 months after the date of index discharge, and every 6 months thereafter until death or the end of follow-up (2 years post-discharge). Patients lost to follow-up were censored at the time of last available contact.
Normally distributed continuous variables were expressed as mean
The study cohort was comprised of 390 patients with HF and MR (mean age 64
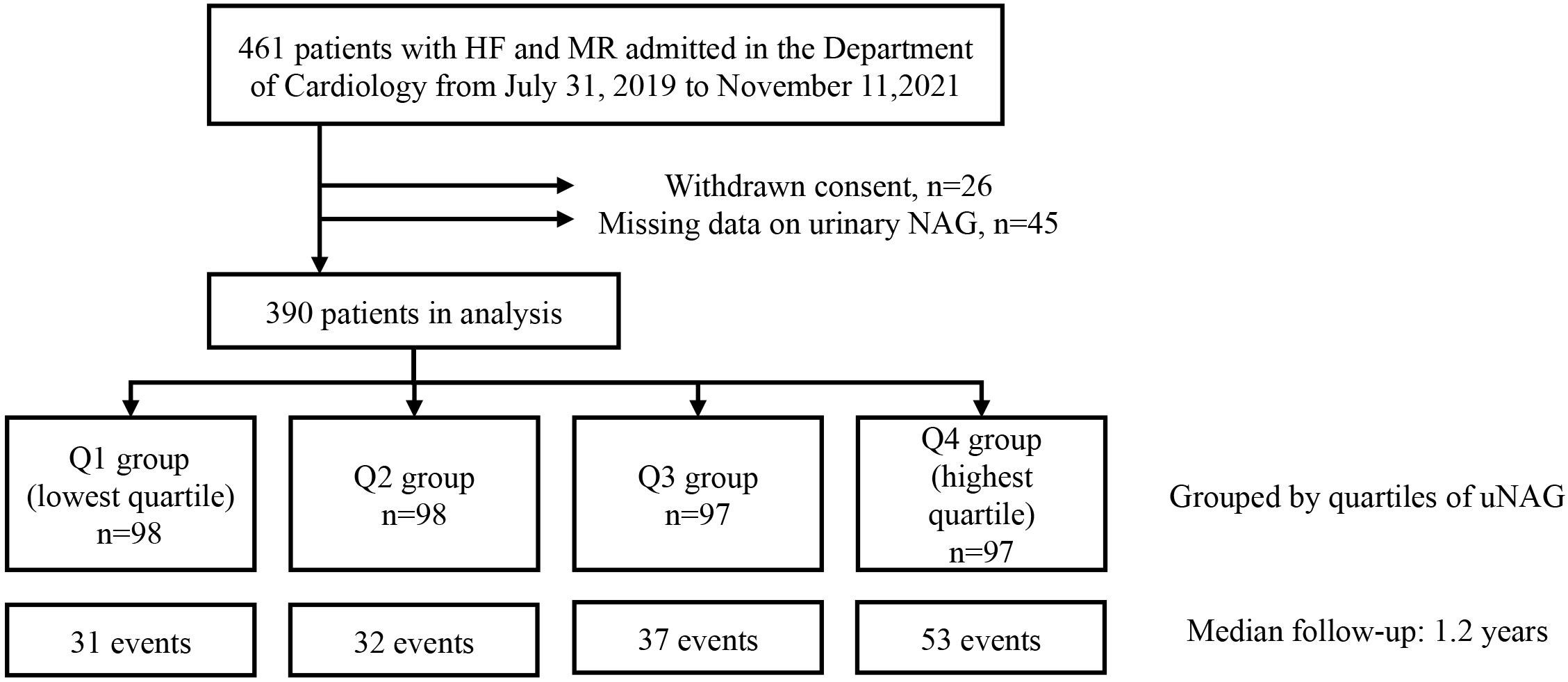
Flow chart of subject selection. Abbreviations: HF, heart
failure; MR, mitral regurgitation; uNAG, urinary
N-acetyl-
Variable | Overall | Q1 ( |
Q2 (4.75 8.08) | Q3 (8.08 13.30) | Q4 ( |
p-value | |
N = 390 | N = 98 | N = 98 | N = 97 | N = 97 | |||
Age, years | 64 |
62 |
65 |
65 |
64 |
0.363 | |
Female, n (%) | 134 (34.4) | 37 (37.8) | 35 (35.7) | 27 (27.8) | 35 (36.1) | 0.468 | |
BMI, kg/m |
23.7 |
24.1 |
23.9 |
23.7 |
23.1 |
0.305 | |
SBP, mmHg | 114.4 |
112.8 |
113.2 |
116.3 |
115.2 |
0.466 | |
DBP, mmHg | 69.1 |
69.4 |
65.9 |
70.6 |
70.6 |
0.050 | |
Heart rate, bpm | 79 |
81 |
77 |
77 |
81 |
0.133 | |
NYHA class | |||||||
I, n (%) | 35 (9.0) | 11 (11.2) | 13 (13.3) | 7 (7.2) | 4 (4.1) | ||
II, n (%) | 240 (61.5) | 71 (72.4) | 63 (64.3) | 58 (59.8) | 48 (49.5) | ||
III, n (%) | 98 (25.1) | 13 (13.3) | 20 (20.4) | 26 (26.8) | 39 (40.2) | ||
IV, n (%) | 17 (4.4) | 3 (3.1) | 2 (2.0) | 6 (6.2) | 6 (6.2) | ||
Comorbidity, n (%) | |||||||
CAD | 127 (32.6) | 26 (26.5) | 33 (33.7) | 36 (37.1) | 32 (33.0) | 0.457 | |
Diabetes | 98 (25.1) | 23 (23.5) | 24 (24.5) | 20 (20.6) | 31 (32.0) | 0.306 | |
Hypertension | 173 (44.4) | 35 (35.7) | 42 (42.9) | 47 (48.5) | 49 (50.5) | 0.157 | |
Atrial fibrillation | 140 (35.9) | 34 (34.7) | 34 (34.7) | 38 (39.2) | 34 (35.1) | 0.895 | |
CKD | 108 (27.7) | 10 (10.2) | 23 (23.5) | 31 (32.0) | 44 (45.4) | ||
Intravenous treatment, n (%) | |||||||
Inotropic agent | 110 (28.2) | 19 (19.4) | 24 (24.5) | 24 (24.7) | 43 (44.3) | ||
Diuretics | 219 (56.2) | 42 (42.9) | 53 (54.1) | 55 (56.7) | 69 (71.1) | 0.001 | |
Vasodilator | 26 (6.7) | 0 (0.0) | 3 (3.1) | 9 (9.3) | 14 (14.4) | ||
Vasopressor | 19 (4.9) | 1 (1.0) | 7 (7.1) | 4 (4.1) | 7 (7.2) | 0.139 | |
Prescriptions at discharge, n (%) | |||||||
ACEI | 13 (3.3) | 4 (4.1) | 4 (4.1) | 5 (5.2) | 0 (0.0) | 0.196 | |
ARB | 21 (5.4) | 5 (5.1) | 7 (7.1) | 2 (2.1) | 7 (7.2) | 0.341 | |
ARNI | 274 (70.3) | 74 (75.5) | 73 (74.5) | 68 (70.1) | 59 (60.8) | 0.099 | |
Beta-blockers | 285 (73.1) | 79 (80.6) | 71 (72.4) | 73 (75.3) | 62 (63.9) | 0.065 | |
MRA | 275 (70.5) | 73 (74.5) | 71 (72.4) | 68 (70.1) | 63 (64.9) | 0.498 | |
Diuretics | 306 (78.5) | 72 (73.5) | 77 (78.6) | 76 (78.4) | 81 (83.5) | 0.406 | |
Laboratory data at admission | |||||||
Hb, mg/dL | 128.7 |
138.4 |
128.3 |
126.9 |
120.9 |
||
NT-proBNP, pg/mL | 1404.0 (657.5, 3707.8) | 850.0 (436.8, 2308.5) | 1058.0 (528.0, 2189.0) | 1518.0 (803.0, 4324.0) | 3754.0 (1569.0, 8704.0) | ||
CRP, mg/dL | 5.9 (5.0, 15.3) | 5.0 (5.0, 8.3) | 5.0 (5.0, 12.0) | 7.5 (5.0, 16.1) | 9.8 (5.0, 28.8) | 0.006 | |
Serum sodium, mmol/L | 139.7 |
139.8 |
140.2 |
140.1 |
138.6 |
0.007 | |
Scr, μmol/L | 84.0 (69.0, 113.0) | 75.0 (66.0, 86.6) | 81.0 (66.3, 105.8) | 97.0 (74.0, 118.0) | 98.0 (78.0, 135.2) | ||
BUN, mmol/L | 7.2 (5.6, 9.9) | 6.7 (5.0, 7.7) | 6.7 (5.5, 8.5) | 7.9 (6.1, 11.4) | 9.1 (6.5, 13.5) | ||
eGFR, mL/min·1.73 m |
82.3 |
97.0 |
88.0 |
74.7 |
69.4 |
||
Urinary microalbumin, mg/g·Cr | 23.5 (12.4, 71.0) | 12.4 (7.6, 23.9) | 21.4 (12.4, 47.1) | 27.0 (16.2, 91.7) | 78.8 (25.0, 246.9) | ||
Echocardiography parameter | |||||||
LVEF, % | 31.9 (25.6, 41.9) | 32.6 (26.1, 42.3) | 34.1 (28.4, 42.5) | 31.9 (25.4, 41.7) | 29.3 (23.9, 41.8) | 0.083 | |
LAD, cm | 4.4 |
4.3 |
4.4 |
4.5 |
4.4 |
0.241 | |
LVEDV, mL | 168.0 |
153.5 |
167.7 |
177.6 |
172.4 |
0.177 | |
LVIDd, cm | 6.1 |
6.0 |
6.1 |
6.3 |
6.1 |
0.261 | |
LVIDs, cm | 5.1 |
5.0 |
5.0 |
5.3 |
5.2 |
0.279 | |
MR grade | 0.136 | ||||||
1, n (%) | 254 (65.1) | 64 (65.3) | 73 (74.5) | 63 (64.9) | 54 (55.7) | ||
2, n (%) | 86 (22.1) | 22 (22.4) | 16 (16.3) | 24 (24.7) | 24 (24.7) | ||
3, n (%) | 26 (6.7) | 8 (8.2) | 5 (5.1) | 6 (6.2) | 7 (7.2) | ||
4, n (%) | 24 (6.2) | 4 (4.1) | 4 (4.1) | 4 (4.1) | 12 (12.4) |
Values are expressed as mean
The results of correlation analyses between uNAG and other clinical variables are presented in Table 2. uNAG levels showed a significant positive correlation with NT-proBNP, CRP and urinary microalbumin. Significant negative correlations were found between uNAG levels and eGFR, Hb, LVEF, and serum sodium.
Variables | Urinary NAG | |
r | p-value | |
Age, years | 0.077 | 0.128 |
Body-mass index, kg/m |
–0.104 | 0.041 |
Systolic blood pressure, mmHg | 0.046 | 0.366 |
Diastolic blood pressure, mmHg | 0.034 | 0.505 |
Heart rate, bpm | 0.007 | 0.892 |
Hemoglobin, mg/dL | –0.198 | |
NT-proBNP, pg/mL | 0.417 | |
C-reactive protein, mg/dL | 0.215 | |
Serum sodium, mmol/L | –0.162 | 0.001 |
Serum creatinine, μmol/L | 0.336 | |
eGFR, mL/min·1.73 m |
–0.338 | |
Urinary microalbumin, mg/g·Cr | 0.496 | |
LVEF, % | –0.116 | 0.022 |
LAD, cm | 0.111 | 0.028 |
LVEDV, mL | 0.106 | 0.061 |
LVIDd, cm | 0.064 | 0.206 |
LVIDs, cm | 0.088 | 0.081 |
Bold font indicates statistical significance. Abbreviations: NT-proBNP,
N-terminal pro-B-type natriuretic peptide; eGFR, estimated glomerular filtration
rate; LVEF, left ventricular ejection fraction; LAD, left atrium dimension;
LVEDV, left ventricular end-diastolic volume; LVIDd, left ventricular internal
diameter in diastolic phase; LVIDs, left ventricular internal diameter in
systolic phase; NAG, N-acetyl-
During the median follow-up of 1.2 years (IQR: 0.4–2.0 years), 3 of the 390
(0.8%) patients were lost to follow-up and 153 (35.5%) experienced a primary
endpoint event (all causes of death (n = 52, 13.3%), HF rehospitalization (n =
126, 32.3%)). Kaplan–Meier analysis revealed that a higher uNAG level
(
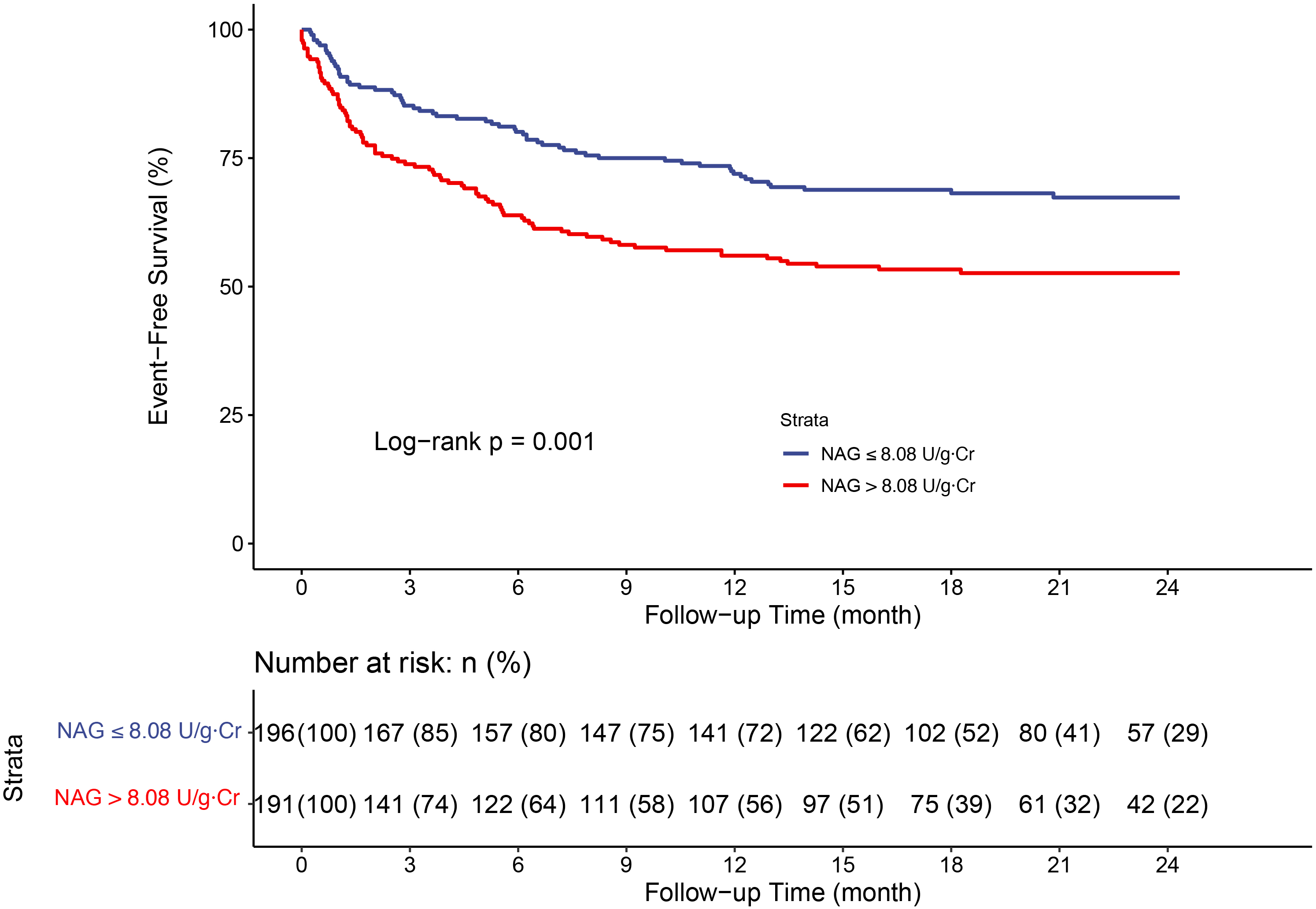
Kaplan–Meier analysis for all-cause death or HF
rehospitalization stratified by urinary NAG median. Abbreviations: NAG,
N-acetyl-
In univariate analysis, higher uNAG level was associated with significantly
increased risks for all-cause mortality, HF rehospitalization, and the composite
of all-cause death or HF rehospitalization (Supplementary Table 1).
Multivariable Cox analysis adjusted for sex, age, CAD, hypertension, diabetes,
CKD, NT-proBNP, LVEF, MR grade, intravenous use of diuretics, and urinary
microalbumin was performed. Each SD (13.80 U/g
Urinary NAG Quantiles | Continuous | ||||
Q1 |
Q2 4.75 |
Q3 8.08 |
Q4 |
Per SD (13.80) greater | |
Events/N at risk | 31/98 | 32/98 | 37/97 | 53/97 | 153/390 |
Unadjusted HR (95% CI) | 1.00 (Ref.) | 1.02 (0.63–1.68) | 1.30 (0.81, 2.10) | 2.19 (1.40–3.41) | 1.31 (1.18–1.45) |
Adjusted HR (95% CI) * | 1.00 (Ref.) | 0.96 (0.58–1.58) | 0.98 (0.60, 1.61) | 1.36 (0.83–2.21) | 1.17 (1.02–1.33) |
* Adjusted for sex, age, CAD, diabetes, hypertension, CKD, NT-proBNP, LVEF, MR
grade, in-hospital use of intravenous diuretics and urinary microalbumin.
Abbreviations: NAG, N-acetyl-
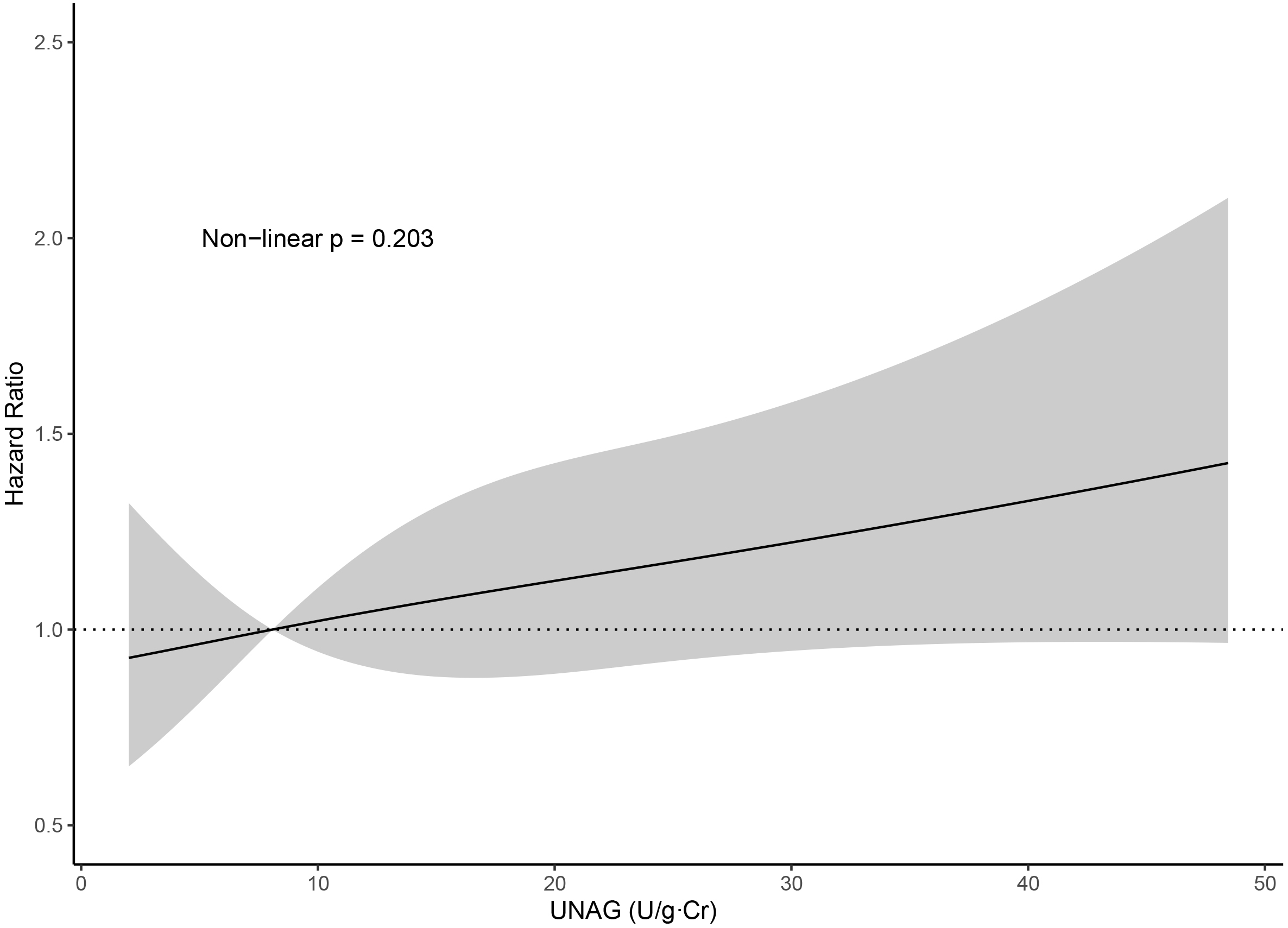
Association between uNAG and all-cause death or HF
rehospitalization, presented as the hazard ratio (solid line) and 95% confidence
intervals (shaded area) and adjusted for sex, age, hypertension, diabetes, CAD,
CKD, NT-proBNP, LVEF, MR grade, intravenous use of diuretics, and urinary
microalbumin. Abbreviations: uNAG, urinary N-acetyl-
Subgroup analyses were performed to determine whether uNAG levels had similar
prognostic value in different populations. Except for the stratification
variable, all analyses were adjusted for sex, age, CAD, hypertension, diabetes,
CKD, NT-proBNP, LVEF, MR grade, intravenous use of diuretics and urinary
microalbumin. As shown in Fig. 4, the association between uNAG and the composite
endpoint was significant only in younger patients, female patients, and in
patients without CAD, diabetes, hypertension, or CKD (all p
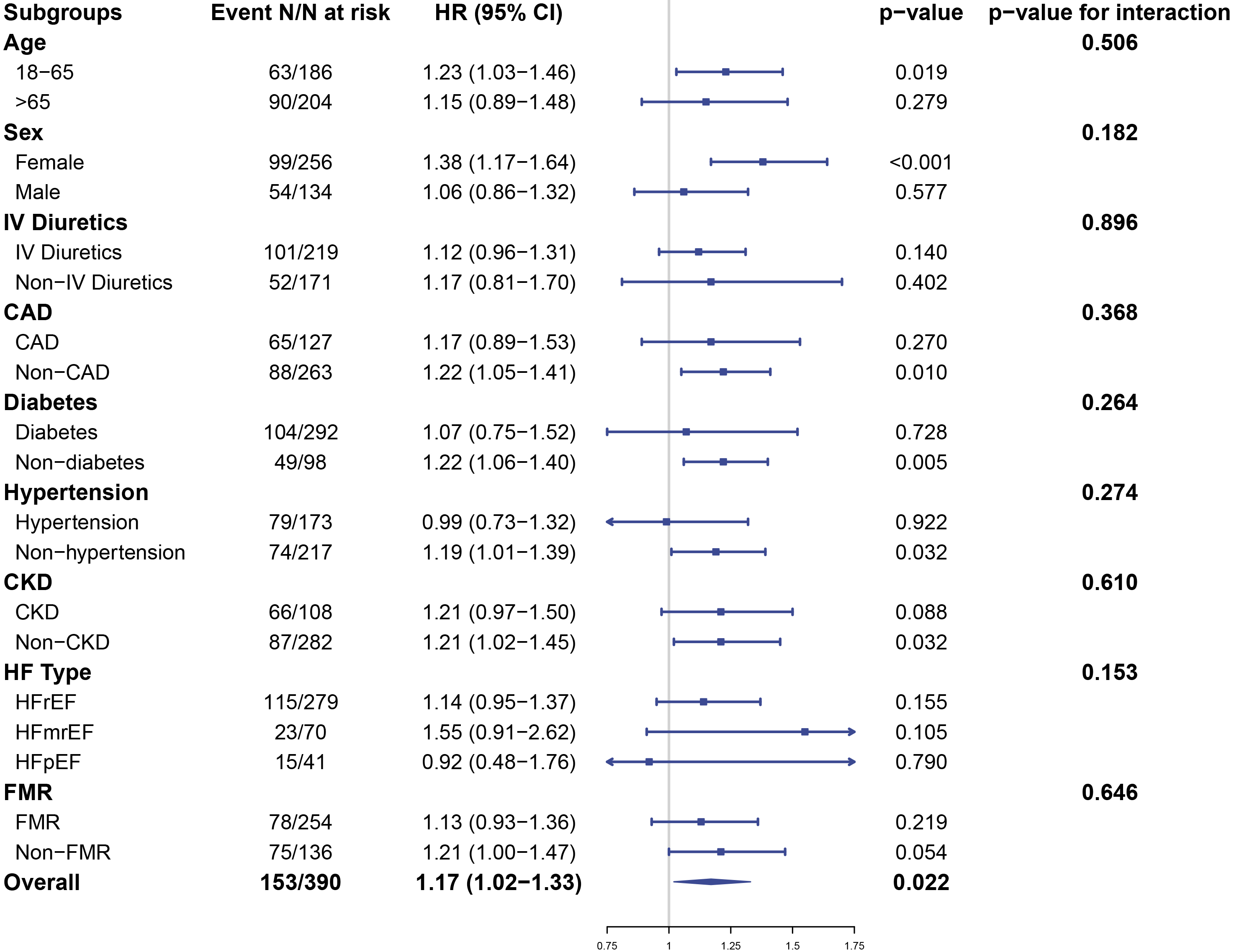
Subgroup analysis showing the hazard ratio for urinary NAG (per
SD: 13.80 U/g
The first major finding of this study was that uNAG levels correlated with the severity of HF. Second, the uNAG level in patients with HF and MR was independently associated with the composite of all-cause mortality or HF rehospitalization, with this association being almost linear. Third, subgroup analysis suggested the uNAG level at admission also had similar prognostic significance in younger patients, and in patients without comorbidities. To our knowledge, this study is the first to evaluate the association between uNAG as a tubular biomarker and adverse outcomes in patients with HF and MR. However, further studies are needed to evaluate the therapeutic implications of this finding.
Renal dysfunction has long been considered one of the factors for poor prognosis
in MR patients. An observational study conducted in 5213 patients who underwent
MitraClip showed that preprocedural renal disease was common (77% with
creatinine clearance
There has been some research into the prognostic value of uNAG for worsening
renal failure and adverse cardiovascular outcomes. Brankovic et al. [34]
showed that an increase in the slope of uNAG levels was associated with a higher
risk of composite endpoint in 263 chronic HF patients, with the association being
stronger than that of plasma creatinine. Damman et al. [35] found that
higher baseline uNAG was the strongest predictor of worse clinical outcome
compared to other tubular markers. In a 10-year follow-up of 149 patients with
chronic HF, Strack et al. [20] used multivariable Cox analysis to show
that uNAG was a significant and independent predictor of all-cause mortality, but
was no longer significant when combined with NT-proBNP. Moreover, longer
follow-up could result in more bias. For example, it is not known whether the
correlation between NT-proBNP and NAG changes over time, and whether or not a
patient’s disease state changes drastically and is restored to the original state
in the interim. In a large cohort of 2466 adults with CKD (eGFR of 20–70 mL/min
per 1.73 m
To our knowledge, this is the first study to investigate the relationship between tubular dysfunction or uNAG and MR. Patients with higher uNAG were found to have a lower baseline hemoglobin level. These patients may be more susceptible to renal tubular injury because of their limited capacity to endure chronic hypoxia. Moreover, there was a trend for higher rates of intravenous diuretic use in the highest uNAG quartile group, indicating the existence of a subclinical venous congestion state and elevated central venous pressure (CVP). Increased CVP has been associated with impaired renal function in patients with advanced HF [38, 39]. Several studies have highlighted the importance of adequate fluid removal and meticulous monitoring of volume status in MR patients. Preload/afterload-reducing medications such as diuretics, nitrates, hydralazines, or ultrafiltration are helpful in reducing the severity of MR [40, 41]. Among patients who underwent a restrictive mitral annuloplasty, those on hemodialysis showed favorable late outcomes compared to those not on hemodialysis [30]. Another study showed that more diuretic use was associated with worse renal function (higher creatinine) and worse prognosis [42]. However, patients with acute decompensated HF treated with diuretics may show increased serum creatinine, but this may simply indicate effective tissue de-edema therapy, which is associated with better outcomes [43]. These findings suggest that the context in which renal dysfunction develops, rather than simply its presence, is the primary determinant of adverse outcomes. Intrarenal physiological changes may be clinically benign and therefore followed with a good prognosis. Further studies are required to elucidate the involved pathophysiology and to further our understanding of cardiorenal syndrome, including the right heart-kidney interaction [44].
A very recent study has suggested that eGFR declines prior to hospitalization for HF, thus highlighting the preadmission period as high-risk and an important opportunity to initiate or up-titrate medications [45]. Monitoring of kidney functions such as the eGFR trajectory may identify patients who are at high risk of clinical deterioration. Serum creatinine (Scr) and creatinine clearance (Ccr) are used to reflect renal injury. However, frequent measurements of Scr can harm patients, while muscle mass, diet and some evidence-based drugs may influence creatinine levels. Hence, more reliable and non-invasive biomarkers are needed. Considering the relative stability and noninvasive testing of uNAG, along with the present study, early recognition of at-risk patients may be achieved by monitoring the trajectory of urinary tubular injury markers.
An meaningful finding of our study was that uNAG was associated with the single endpoint of HF rehospitalization. Advanced HF was characterized by worsening symptoms, recurrent hospitalizations, and greater lengths of hospital stay, incurring significant financial burdens to the patient and the healthcare system. Heidenreich et al. [46] estimated that hospitalization for HF would account for 80% of the cost for care of HF patients. In China, the inpatient cost among urban HF patients accounted for 66% of their total cost [47]. The economic implications of rehospitalizations are self-evident, and substantial savings in healthcare system will be achieved if we can reduce HF admission rate. Our observation that uNAG played a role in predicting rehospitalizations emphasized the possibility of uNAG being used as a prognostic marker. Urine-based biomarker monitoring in clinical practice is repeatable and cost-effective. Given the large number of patients with HF and MR and the ease and low cost of urine sample collection and analysis, monitoring uNAG for early identification and outpatient intervention of high-risk patients should lead to improved outcomes as well as reduced health expenditure.
So far, the effects of percutaneous therapy on the MR population have given opposite, and yet complementary results [48, 49]. Following in-depth analysis and comparison, it was concluded that appropriately selected patients (disproportionate severe MR with cardiac function) may benefit from percutaneous therapy. In light of our finding that admission uNAG level was an independent prognostic factor for patients with MR, this raises the question of whether baseline clinical test indicators could improve candidate selection for intervention. Unfortunately, research in this area is still sparse and our study was mainly hypothesis generating in nature. More studies are needed to confirm our conclusions and to elucidate the cause-effect relationship for higher uNAG levels being associated with poorer prognosis, as well as whether tubular dysfunction could be a potential target for MR therapeutics.
In summary, this study has advanced our understanding of cardiorenal interactions in MR, its impact on patient manifestations during hospitalization, and on the clinical outcomes after discharge. Confirmation of the link between tubular dysfunction (as indicated by urinary NAG levels) and MR will give physicians a cheap and non-invasive biomarker to facilitate decision making and reduce healthcare costs.
There are several limitations to this study. First, extrapolation of the results are limited by the single-center and observational nature of the study. The relatively small sample size might also have introduced selection bias. Second, the AUC value of NAG was relatively low. We speculate that the preliminary nature of this work may limit the power to make robust conclusions but lays the foundation for future studies. We believed that studies with a larger sample size and longer follow-up may achieve superior predictive accuracy. Third, the improvement in the C-index was small and we therefore had insufficient power to demonstrate the additional prognostic value of NAG levels in this cohort, particularly in comparison to NT-pro BNP. Given the multifactorial nature and complex pathophysiology of MR, it may be that the prognosis of patients with MR is also dependent upon other clinical characteristics (e.g., the duration of illness, baseline cardiac function) rather than solely renal function, including tubular function. Our study was not designed to generate a prognostic model for use, but merely to explore the association between urinary NAG levels and the risk of adverse events in patients with MR. After adjustment of multiple confounders, NAG remained an independent predictor of HF rehospitalization and the composite endpoint of HF rehospitalization and death. Nonetheless, the predictive value of NAG for MR risk stratification purposes needs to be formally assessed and our findings need to be replicated in a different cohorts before these could be applied in clinical practice. Fourth, the relationship between uNAG and volume status could not be assessed because right heart catheterization was not performed during inpatient treatment. Furthermore, it was not known whether uNAG values were affected by the use of diuretics prior to hospitalization. Fifth, urinary NAG levels may fluctuate with disease progression and treatment application. A single measurement upon admission may therefore fail to track longitudinal changes and hence misrepresent its prognostic significance. Finally, a longer follow-up period should provide more accurate and complete information on the prognostic significance of uNAG.
We demonstrated that higher urinary NAG levels in patients with HF and MR can independently predict the risk of all-cause death or HF rehospitalization. These findings suggest that the uNAG level at admission may be a novel prognostic factor in patients with HF and MR.
MR, mitral regurgitation; HF, heart failure; uNAG, urinary
N-acetyl-
The datasets analyzed during the current study are available from the corresponding author on reasonable request.
TZ, GC, SZ, CZ, CJ, YX and MX were responsible for the study design and execution. CZ finished the echocardiography analysis. GC collected and cleaned the data. TZ, GC and SZ performed the data analysis. TZ and SZ wrote the manuscript. YX and MX edited and reviewed the paper. All authors contributed to editorial changes in the manuscript. All authors read and approved the final manuscript.
The study was conducted in accordance with the Declaration of Helsinki and approved by the Institutional Review Board of the Second Affiliated Hospital, Zhejiang University School of Medicine (0039-2022). Written informed consent has been obtained from all patients to participate in the study.
The authors wish to express their gratitude for the help and support provided by the staff of the Second Affiliated Hospital, Zhejiang University School of Medicine.
This research was funded by the Key Research and Development Project of Department of Science and Technology of Zhejiang Province, grant number 2020C03118 to M.X., Provincial and Ministry Joint Major Projects of National Health Commission of China, grant number WKJZJ-1703 to M.X.
The authors declare no conflict of interest.
Publisher’s Note: IMR Press stays neutral with regard to jurisdictional claims in published maps and institutional affiliations.