- Academic Editors
†These authors contributed equally.
Background: Chronic kidney disease (CKD) burden is crucial both on a global scale and at individual patient level, affecting morbidity and mortality directly and through its effect on both cardiovascular damage and CKD progression to end-stage-kidney-disease (ESKD). Unfortunately, the awareness of CKD is poor, with few CKD patients conscious of the severity of their health status. The principal biomarker of kidney function is estimated glomerular filtration rate (eGFR). Methods: We searched the literature and present a review article with the aim of summarizing the role of eGFR in clinical research. In particular, we report the eGFR role as a prognostic, enrichment and endpoint biomarker and its role in the early detection of CKD. Results: eGFR has a major role as a biomarker in clinical research. As a prognostic marker, eGFR reduction is associated with cardiovascular events, ESKD and mortality. As an enrichment biomarker, eGFR values are pivotal for selecting patients to be included in randomized and observational studies; it helps to test a pre-defined drug in early CKD or in more advanced CKD allowing also to avoid screening failures and to shorten the duration of clinical trials. Moreover, eGFR decline (expressed as a percentage of reduction from baseline or continuous slope) can be considered a good endpoint in clinic trials overcoming delays whilst waiting for hard endpoints to develop. Conclusions: eGFR is a strong clinical measure for both observational and intervention studies. It is also helpful in screening the general population for kidney disease and, in particular, to increase awareness of CKD.
Chronic kidney disease (CKD) is defined as abnormalities of kidney structure or function, present for at least 3 months, with implications for health. Chronic kidney disease is classified based on cause, estimated glomerular filtration rate (eGFR) category (G1–G5), and albuminuria category (A1–A3) [1]. Incidence and prevalence of CKD vary among countries and are mainly influenced by ethnicity and socioeconomic status. The worldwide prevalence of CKD is 13.4% (11.7–15.1%), with thousands of patients (range between 4.902 and 7.083 million) requiring renal replacement therapy (RRT, or also known as end-stage-kidney-disease, ESKD) [2]. Projections from the Global Health Observatory suggest that mortality due to CKD will reach an impressive rate of 14 per 100,000 people by 2030 [3]. The burden of CKD is relevant on a global scale but also at from the perspective of individual patientss, affecting morbidity and mortality directly, and through its effect on both cardiovascular (CV) damage and CKD progression to ESKD. Moreover, the epidemiology trend showed that CKD prevalence and incidence have doubled in the past three decades, increasing by 87% and 89% from 1990 to 2016, respectively [4]. These data gain significance if considering that the awareness of CKD is poor, with only a few CKD patients, less than half, conscious of the severity of their health status [5]. In addition to the epidemiological perspective, it is important to remark that the presence of CKD is, per se, associated with an increased risk for CV events, all-cause death and kidney disease progression [6, 7]. All these data are alarming and prompt the need for further effort in the attempt of preventing, or at least relenting, the future trend, and improving individual prognosis. To this aim, the principal strategy that has been advocated is to intensify research in terms of detection of CKD, risk stratification of CKD patients and improving care of these patients [8]. The principal biomarker of kidney function level is represented by the eGFR. The acronym GFR refers to a measure of the sum of the filtration rates of all functioning nephrons, which can be measured or estimated, and which is used in clinical practice to diagnose chronic kidney disease, determine its degree of severity, and establish the prognosis of a patient with CKD, which is also helpful for therapeutic decisions [9]. Similarly, eGFR is widely used in clinical research to select patients to include in clinical trials (enrichment biomarker), to monitor the treatment effect (endpoint biomarker), to predict the progression to ESKD in observational studies (prognostic biomarker). Herein, we present a narrative review, which summarizes the clinical and research contexts in which eGFR is used. With respect to clinical use, we will also present a brief discussion of the central role of eGFR in screening patients with CKD.
The article search for this review was performed in PubMed and was according to
author knowledge and experience on the specific field of eGFR. The glomeruli
filters approximately 180 liters (L) of plasma per day which corresponds to 125
milliliters/minute (mL/min) of glomerular filtration. Normal GFR values, which
are related to age, sex, and body size, are approximately 130 mL/min/1.73 m
In recent years other biomarkers like
The main equations used to estimate eGFR through creatinine levels are the modification of diet in renal disease (MDRD) and the Chronic Kidney Disease Epidemiology Collaboration (CKD-EPI) equation.
The MDRD equation estimates eGFR adjusted for the body surface, age, gender,
serum creatinine and race. The estimation equation is GFR = 186
The major limit of the MDRD equation is to underestimate the GFR at higher
ranges of kidney function [19]. The 2009 CKD-EPI equation, also based on serum
creatinine, was developed with the aim of formulating an equation as accurate as
the MDRD at GFR less than 60 mL/min/1.73 m
Both the MDRD and the 2009 CKD-EPI place importance on the Black race because
previous studies indicated a higher average serum creatinine level for the same
measured GFR level in Black participants than in non-Black ones [21]. However, it
has been shown that the difference in terms of race has more of a cultural basis
than a biological one [22]. From these assumptions, a new CKD-EPI equation was
reformulated in 2021 and did not include race in the GFR assessment [23, 24]. The
new estimated equation is eGFRcr = 142
Historically, the association between CKD and increased CV risk has been related to the presence of comorbidities such as hypertension and diabetes, which are per se traditional CV risk factors. Furthermore, the combination of electrolyte abnormalities, anemia, as well as the increase of blood urea are some of the additional factors that contribute to the CV burden in these high- risk patients.
However, several studies have demonstrated that eGFR acts as a strong predictor of CV events (mainly coronary heart disease, chronic heart failure, stroke, peripheral vascular diseases, CV death), regardless of the presence of any other comorbidities and clinical or demographic variables such as age or gender. Moreover, such an association is present in patients with already assessed CKD, namely those already under the care of a nephrologist, but also in subjects derived from the general population [6]. Large studies including CKD patients showed that considering 100 mL/min as a reference point for a low eGFR , the risk of CV fatal and non-fatal events was almost doubled [31]. In a cohort of CKD patients followed up by nephrologists in 40 Italian centers, the incident rate of fatal and non-fatal events over time was progressively higher moving from CKD stage 1–2 to 5, with a relative risk of 48% moving from one stage to the next more severe stage (Fig. 1) [32].
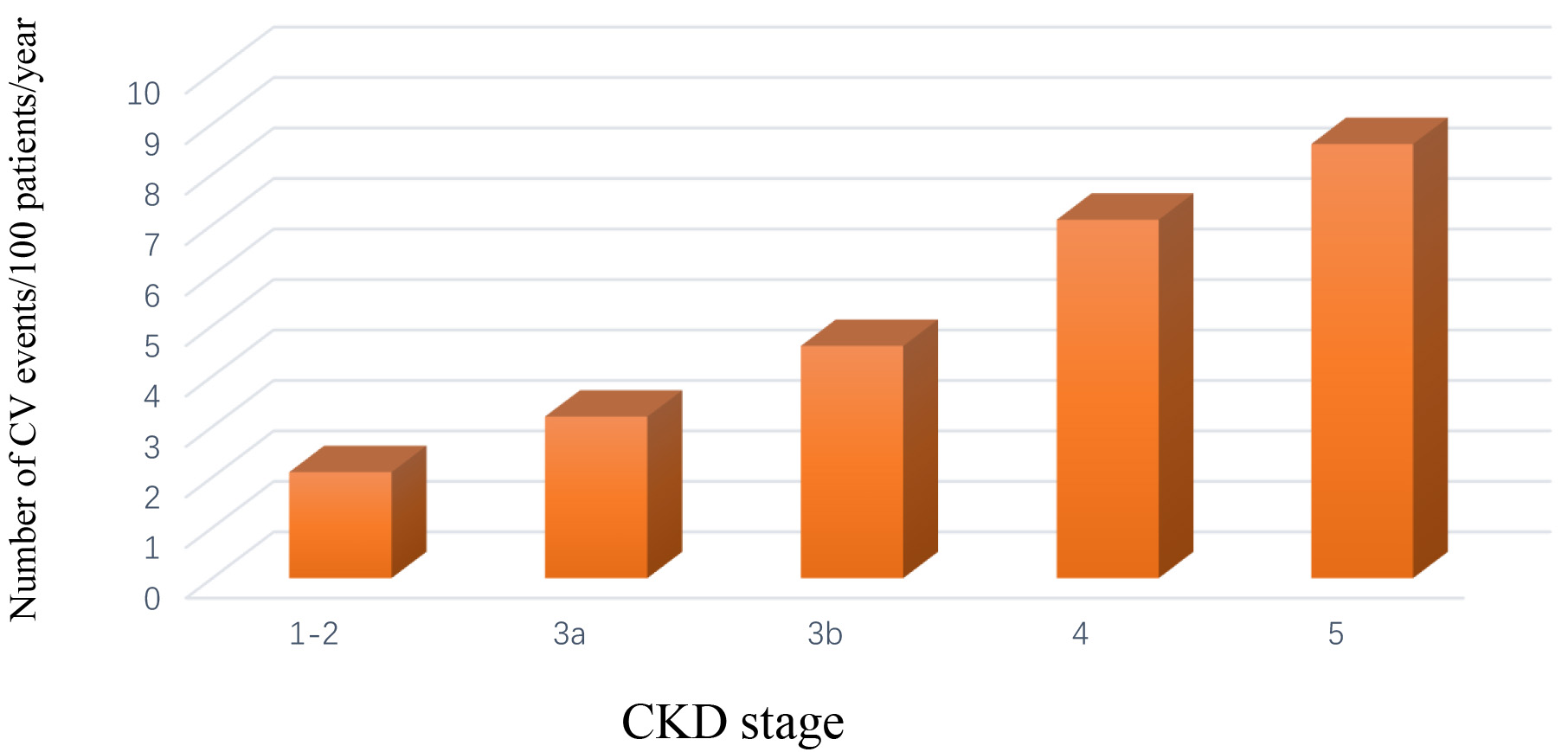
Rate of fatal and non-fatal cardiovascular events (myocardial infarction, stroke, heart failure, peripheral vascular disease) in chronic kidney disease patients stratified by stage [32].
In a recent meta-analysis which enrolled around 10,000 individuals from the
general population, the independent association between eGFR reduction and future
CV events, regardless of previous history of CV disease was demonstrated.
Particularly, a higher risk of CV death is evident in patients with eGFR level
From a prognostic perspective, the current risk scores including traditional
risk factors only (age, blood pressure, low density lipoprotein (LDL)
cholesterol, smoking habit and gender) underestimate CV risk in CKD cohorts [35].
Overall, the inclusion of the two “kidney measures”, eGFR and albuminuria,
significantly improve CV risk prediction both in the general population and in
high risk patients such as CKD patients [35]. Besides the traditional risk
factors, inactivity may also be an important predictor of mortality as there have
been studies linking CKD with low exercise levels as well as improved hazard
ratios in patients who did exercise [36, 37]. The Gruppo di Lavoro Italiano
Sarcopenia-Trattamento E Nutrizione (GLISTEN) study highlighted the connection
between eGFR and mortality in older patients, with the highest being in patients
with an eGFR
Unfortunately, the exact mechanism by which eGFR decline increases risk of CV risk is only partially understood.
Several factors may play a crucial role. For instance, the imbalances in matrix
metalloproteinases (MMPs) in CKD patients have been associated with profibrotic
and pro-inflammatory mechanisms with subsequent structural changes that lead to
atherosclerotic plaque maturation along with arterial remodeling [39]. This
results in an increased risk of arterial wall pathologies in CKD patients such as
aneurism complications and atherosclerotic disease. Furthermore, the eGFR
reduction in CKD patients is associated with a persistent, low-grade inflammation
which correlates with increased CV risk. Different factors such as the decreased
elimination of cytokines, metabolic acidosis, as well as the oxidative stress and
the recurrence of infections contribute to the inflammatory status that
characterizes CKD patients [40]. Moreover, studies have shown that these changes
contribute to the development of heart failure with preserved ejection fraction
(HFpEF) in patients with CKD [41]. It was also possible to detect a specific set
of biomarkers which predict all-cause and CV mortality with more accuracy than
other biomarkers: increased values of interleukine (IL)-6 are a stronger
predictor than other cytokines such as tumor necrosis factor (TNF)-
Further investigations are needed to better understand the link between eGFR decline and the increased CV risk.
The evaluation of kidney function is also important from an interventional perspective, namely when considering eGFR decline as a modifiable (by therapies) risk factor. Different interventional studies such as those aiming to reduce blood pressure levels have shown benefits in terms of both renal and CV risk due to a slower rate of eGFR decline. This is the case with renin-angiotensin-aldosterone-system inhibitors (RAAS-I): these drugs warranted a reduction in relative risk of CV events of 22% in the ramipril-treated group, compared to the placebo-treated group, in the heart outcomes protection evaluation (HOPE) study [47].
In the past few decades, new promising drugs have been developed and approved for CV risk reduction in CKD patients, particularly the sodium-glucose cotransporter 2 inhibitors (SGLT2-is) and novel non-steroidal mineralocorticoid receptor antagonists (MRAs). The SGLT2-is act by reducing the reabsorption of glucose in the renal proximal tubule [48, 49, 50]. The Canagliflozin Cardiovascular Assessment Study (CANVAS) reported the significant benefit of the SGLT2-i canagliflozin, in reducing the composite outcome (CV death, nonfatal myocardial infarction or stroke) by about 15% in patients suffering from type 2 diabetes (DM2) with elevated CV risk both for primary and secondary prevention [51].
Another paramount study is the EMPA-REG OUTCOME trial (Empagliflozin
Cardiovascular Outcome Event Trial in Type 2 Diabetes Mellitus Patients) where
SGLT2-i empagliflozin was shown to reduce CV morbidity and mortality in DM2
patients with eGFR
A recent meta-analysis of the Empagliflozin Outcome Trial in Patients With Chronic Heart Failure With Reduced Ejection Fraction (EMPEROR)-reduced and the Dapagliflozin and Prevention of Adverse Outcomes in Heart Failure (DAPA-HF) trials showed that SGLT2-i empagliflozin and dapagliflozin respectively, are associated with a 14% reduction in CV death in patients with reduced ejection fraction with or without diabetes (pooled HR: 0.86, 95% CI: 0.76–0.98; p = 0.027). Moreover, SGLT2-i treatment was associated with a 26% relative risk reduction of the combined outcome of CV death or first hospitalization for heart failure, and with a 25% decline of the composite outcome of recurrent hospitalizations for heart failure or CV death. The risk of the composite renal endpoint was also reduced (HR: 0.62, 95% CI: 0.43–0.90; p = 0.013) [53].
The novel MRAs have also been shown to improve CV prognosis in CKD patients. Mineralocorticoid receptor (MR) is overactivated in both CKD and heat failure (HF) with a subsequent increased expression of inflammation and fibrotic pathways, which lead to organ injury [54]. As a protagonist of two important studies, Finerenone in Reducing Kidney Failure and Disease Progression in Diabetic Kidney Disease (FIDELIO) and Finerenone in Reducing Cardiovascular Mortality and Morbidity in Diabetic Kidney Disease (FIGARO), as well as their combined analysis (FIDELITY), the novel MRA finerenone has shown, in patients with CKD and DM2, not only to improve renal function but also to reduce CV risk, with lower incidence of hyperkalemia, as compared to steroidal MRA, albeit confirming pro-inflammatory and profibrogenic pathways [55, 56, 57]. The Finerenone in Reducing Kidney Failure and Disease Progression in Diabetic Kidney Disease (FIDELIO-DKD) double-blind trial, which included 5734 patients with both DM2 and CKD, showed a significant effect of finerenone in reducing the progression of CKD and CV event rate [58]. Moreover, in the Finerenone in Reducing Cardiovascular Mortality and Morbidity in Diabetic Kidney Disease (FIGARO-DKD) trial, treatment with finerenone, compared to placebo, was shown to reduce new-onset HF (1.9% versus 2.8%; HR: 0.68, 95% CI: 0.50–0.93; p = 0.0162) and to ameliorate HF outcomes in patients with DM2 and CKD, regardless a previous history of HF [59]. The crucial point of discussion is that the CV risk reduction in almost all these studies was present, especially in patients who showed a lower eGFR decline after the commencement of treatment.
Besides the association of eGFR with CV risk previously discussed, this marker
also has a pivotal role in predicting other important outcomes such as CKD
progression and all-cause mortality [6]. The association between increased
mortality risk and reduced renal function may also in part be explained by the
inflammatory milieu of CKD, characterized by oxidative stress, vascular damage
and endothelial dysfunction which lead to an increase in global mortality risk
and progression of kidney damage [60, 61]. An even more interesting finding is
the association between increased eGFR variability and mortality rate, especially
when compared to a stable kidney function. In this regard, Turin et al.
[62] demonstrated that mortality rates were highest for people with an increase
in eGFR of 5 mL/min/1.73 m
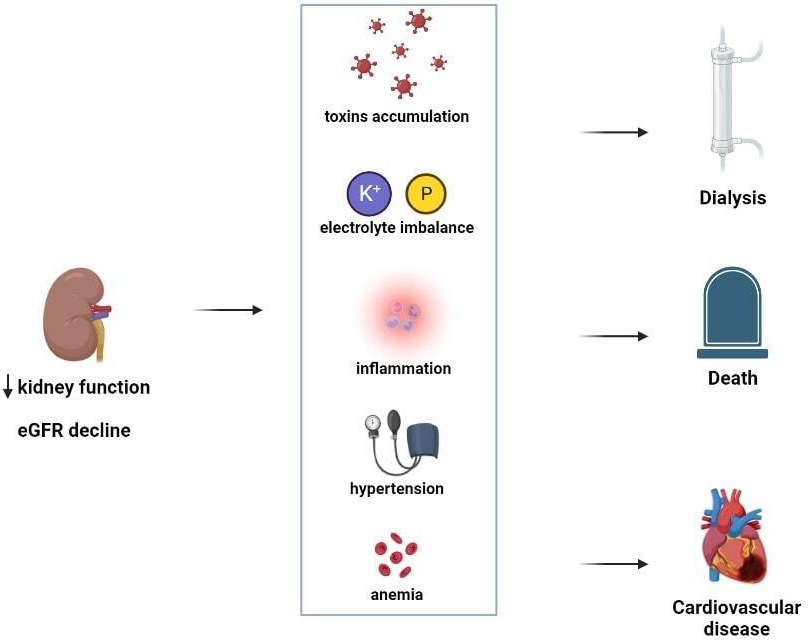
Estimated estimated glomerular filtration rate (eGFR) and its association with several pathophysiologic mechanisms. Estimated GFR reduction is associated with the development of several comorbidities (increase in blood toxin levels, electrolyte imbalances such hyperkalaemia and hyposodiemia, inflammation, arterial hypertension and anemia) that, taken together, dramatically increase the risk for future events.
The selection of patients to be included in randomized studies is a challenging
topic since clinical trials with novel drugs may in one sense reflect the
clinical practice world and be applicable in such direction. Normally, one
strategy to select patient is based on the levels of certain biomarkers, the
so-called biomarker enrichment [67]. Levels of kidney function are detrimental to
select patients to be included in randomized studies. The first big trials
demonstrating the protective effect of RAAS inhibitors on CKD progression, such
as the Reduction of Endpoints in NIDDM (non-insulin-dependent diabetes mellitus) with the Angiotensin II Antagonist
Losartan (RENAAL) and Irbesartan Diabetic Nephropathy Trial (IDNT) studies, used
serum creatinine as enrichment biomarker [68]. Subsequently, creatinine has been
replaced by eGFR levels to the same aim, which may help to account for
differences in gender, as the eGFR formulas have a correction factor for gender.
Moreover, when using eGFR, a pre-defined drug can be tested in early CKD (e.g.,
eGFR
An endpoint of a specific study is a measure (also called event) that registers
a significant change in the quality of life or, in most negative and unfortunate
scenarios, the end of life. A sufficient number of endpoints is mandatory to have
a good study power and answer the study question appropriately, in both
observational and interventional studies. In this context, eGFR is considered a
measure of kidney function decline over time and therefore works as an endpoint,
modeled in different mathematic forms. In observational studies, using eGFR as an
endpoint is commonly reported as the percentage of eGFR decline from baseline
evaluation (e.g., 30%, 40%, 57% eGFR decline) or continuous eGFR slope. In
both cases, eGFR endpoints are a surrogate of another major end-point, namely
ESKD. The advantage of using eGFR decline as an endpoint is the fact that hard
endpoints such as mortality, CV events and ESKD need many years, often decades,
to develop. Hence, having more events in a brief period of a certain endpoint
which can be considered a surrogate of these other major events is instrumental
in clinical research. Several previous studies showed that reductions of less
than 57% in eGFR values over time can be used as alternative endpoints for
evaluating the progression of CKD. A study conducted on individual meta-analysis
data of 1.7 million participants from 35 cohorts in the Chronic Renal Failure
Prognosis Consortium evaluated the possibility of using less than 57% reductions
in eGFR values over a period of two years to predict the development of ESKD
[72]. Furthermore, since many CKD patients die before reaching ESKD, the
associated mortality risk has also been assessed. The hazard ratios of ESKD
and mortality were higher for larger reductions in eGFR. The 10-year mean risk of
ESKD was also calculated in patients with a baseline eGFR value of 35 mL/min/1.73
m
Several landmark studies in the field of nephrology from recent years have used both the percentage eGFR reduction as well as continuous eGFR slope to demonstrate the response to nephron-protective therapies. In a detailed analysis of the EMPA-REG-OUTCOME trial, the presented eGFR slope showed that empagliflozin has the potential to slow the decline of eGFR after treatment for around three years, even in patients at higher risk of worsening CKD [76]. Another work that has supported the eGFR slope as a possible future endpoint in chronic kidney disease progression studies is a study conducted in patients with DM2 being treated with Canagliflozin [77]. Most of the novel trials, testing the efficacy of endothelin receptor antagonists, mineralocorticoid receptor antagonists or SGLT2 inhibitors reported the analysis of treatment effect on eGFR slope [72, 78, 79]. The percentage of eGFR decline has been also evaluated as an outcome of interventional studies. 40% and 57% eGFR reduction from baseline (start of treatment visit) are considered good endpoints of response to treatment, even in clinical scenarios of a rapid, yet only functional, eGFR decline immediately after the treatment initiation [71, 79]. Interestingly, data from the FIGARO-DKD study suggests caution in interpreting the 40% eGFR decline. In fact, the effect of finerenone in this trial was significant when tested on 57% eGFR reduction and not significant (borderline confidence interval) on 40% eGFR decline suggesting that potentially in some occasions, more powerful (and not more frequent!) events may reveal treatment effects more clearly. These alternative endpoints are also useful in randomized studies for enrolling patients at early stages of kidney disease, where hard endpoints occur after many years and therefore surrogate endpoints are needed. On the other hand, testing the efficacy of nephroprotection at an early stage of CKD is the main aim of clinical research. The role of eGFR for clinical and research purposes has been already developed in several review articles as reported in Table 1 [9, 80, 81, 82, 83, 84, 85, 86, 87], whereas the main contents and novelties of the present review are repored in Table 2 (Ref. [6, 60, 62, 63, 64, 68]).
Article | First author | Main concept |
Assessment of Glomerular Filtration Rate in Health and Disease: A State of the Art Review [80] | A.S. Levey & L.A. Inker | GFR is the main index of kidney function. It has a paramount role in the kidney disease care and in drug dosing. GFR should be estimated using the most accurate GFR estimating equation, particularly the CKD-EPI based on creatinine or Cystatin C. |
Evaluating the Performance of GFR Estimating Equations [81] | L. A. Stevens | Estimated GFR represent a valid tool in the management of CKD. Despite the limitations related to the lack of a single eGFR equation that applies to all people, eGFR equations are crucial in clinical research and healthy policy related to CKD. |
GFR Estimation: From Physiology to Public Health [82] | A.S. Levey | eGFR equations are less subjected to risk of bias when used in CKD patients compared to the general population. Further improvement in eGFR equations are needed in order to better represent differences in populations, to use multiple filtration markers, and to use statistical techniques to compare eGFR to measured GFR. |
Measured and estimated glomerular filtration rate: current status and future directions [83] | A.S. Levey | GFR is considered the main marker of kidney function. Estimated GFR (eGFR) and measured GFR (mGFR) are both associated with errors compared to the actual GFR (measured GFR, mGFR). While further adjustment of the GFR calculation equations is required, eGFR is recommended for initial filtrate assessment, with mGFR generally considered an important confirmation test. |
Measurement and Estimation of GFR for Use in Clinical Practice: Core Curriculum 2021 [9] | L.A. Inker & S. Titan | The GFR is used in clinical practice and research as the main tool for diagnosing, staging and managing CKD as well as for defining CKD-related prognosis and mortality risk. GFR is estimated using equations using the serum creatinine level. However accurate, GFR estimation may require confirmatory tests of which currently available include equations based on Cystatin C, urinary or plasma clearance of markers of exogenous filtration or urinary creatinine clearance. |
New and old GFR equations: a European perspective [84] | P. Delanaye | GFR is considered a cornerstone tool in CKD. However, all the equations available for calculating GFR give a rough estimate rather than the actual value. A precise measurement of GFR in specific populations and/or specific clinical situations might be required. |
Determining the Glomerular Filtration Rate—An Overview [85] | E. Schaeffner | The GFR determination is crucial in clinical practice as it helps, among other things, in therapeutic decisions. GFR can be calculated or more commonly estimated using several formulas. The most used filtration marker to date is creatinine, although recently Cystatin C is taking on an increasingly important role. Furthermore, new formulas for GFR evaluation, applicable to all ages, are emerging. |
Estimated Glomerular Filtration Rate in Chronic Kidney Disease: A Critical Review of Estimate-Based Predictions of Individual Outcomes in Kidney Disease [86] | L. Zsom | GFR assessment is a useful tool in clinical practice being also used as a prognostic indicator of chronic kidney disease progression. However, it should be considered as an initial screening tool, since its reduction over time should be evaluated overall together with the general clinical context. |
Estimating glomerular filtration rate: is it good enough? And is it time to move on? [87] | D. P. Murphy | The evaluation of GFR is fundamental in daily clinical practice. Several studies have demonstrated the non-superiority of GFR measurement compared to its estimation using formulas in predicting renal outcome. |
(e)GFR, (estimated) glomerular filtration rate; CKD, chronic kidney disease; CKD-EPI, Chronic Kidney Disease Epidemiology Collaboration.
eGFR as | Key-concepts |
Prognostic biomarker | - The eGFR variability is associated with higher risk of all-cause death and CV events [62]. |
- Estimated GFR decline is a strong predictor of CKD progression (particularly ESKD), CV events and mortality [60]. | |
- Even mild or moderate reductions in eGFR have potentially important clinical sequelae [6]. | |
- The cardiorenal risk related to eGFR declines is impaired by the presence of CKD comorbities such as hyperkalemia, hyperparathyroidism, increased serum phosphate levels, dyslipidemia, metabolic acidosis and hyperuricemia [68]. | |
Enrichment biomarker | - Kidney function values are detrimental to select patients to be included in randomized and observaitonal studies [64]. |
- The evaluation of eGFR values not only allows a pre-defined drug to be tested in early CKD or in more advanced CKD but also it helps to avoid screening failures and to shorten the clinical trials duration [63]. | |
- The eGFR trajectory before study initiation (pre-trial eGFR slope) may inform about the treatment effect and thus it can be considered an important enrichment criterion in future research. | |
Endpoint biomarker | - Estimated GFR decline (expressed as percentage of reduction from baseline or continuous slope) can be considered a good endpoint in clinic trials. |
- The advantage of using eGFR decline as endpoint is the fact that the hard endpoints need many years, often decades, to develop. | |
- The continuous eGFR slope is considered an accurate measure of eGFR changes over time since it encompasses severel eGFR values over time and it also may account for the presence of other confounding variables. |
(e)GFR, (estimated) glomerular filtration rate; CKD, chronic kidney disease; ESKD, end-stage-kidney-disease; CV, cardiovascular.
More than 850 million people worldwide have CKD and by 2040 CKD is predicted to be the fifth most prevalent chronic condition in the world [88, 89]. Although the number of individuals affected by CKD is so high, only one in three patients with CKD get diagnosed [90]. This can be attributed to the fact that CKD, especially in early stages is a silent disease, meaning most patients remain asymptomatic until the disease progresses to advanced stages [91]. Another important factor is that there is no standardized systematic screening and treatment strategy for CKD. Moreover, CKD is often viewed as a complication of diabetes mellitus or hypertension but not as a disease itself. As all of this leads to CKD being underdiagnosed, the referral to a nephrologist often does not happen until it is too late and CKD advances to the later stages, where the initiation of dialysis remains inevitable [92]. Further prompting the need to diagnose CKD earlier and prevent disease progression is the established fact that dialysis, although improvement has been made in the last decades, is still associated with incredibly high mortality rates of 10 to 20 times greater than the general population and consumes 5–7% of total health care budgets [86].
The Kidney Disease: Improving Global Outcomes (KDIGO) guidelines define CKD as a decreased eGFR (
According to the WHO, principles for screening of a disease include the disease being an important public health problem affecting a large group of individuals, availability of a suitable test or examination as well as treatment options [97]. All those criteria are met with CKD as it is even sometimes described as a global epidemic, eGFR and albuminuria testing is cost effective and treatment options are available [98, 99, 100]. Especially in the last couple of years, with the results from large scale studies showing that SGLT2 inhibitors or nonsteroidal mineralocorticoid receptor antagonists (nsMRA) additional to the already established angiotensin-converting enzyme inhibitors (ACEi) and sartans can reduce mortality and slow progression of CKD, the focus in nephrology has shifted from replacing the kidney via dialysis to actually preventing ESKD [99].
In order to fulfill this goal and to increase the number of patients diagnosed
with CKD, the awareness of CKD as a global burden has to rise [100]. The first
question that must be answered in this context is, which individuals should be
screened for CKD. The KDIGO guidelines on CKD from 2012 omit the screening for
CKD completely, which is why in 2021 a KDIGO controversies conference was held to
help answer that question [90]. In this conference, risk groups were defined
which should be screened by general practitioners or doctors from other
specialities, as screening for CKD seldom happens by a nephrologist. The defined
risk groups primarily included patients with diabetes, hypertension, or CV
disease as well as patients with obesity, family history of renal disease, acute
kidney injury (AKI) in patient history, older age, and other high-risk
comorbidities. As stated above, in those individuals an assessment of the
glomerular filtration by eGFR calculation as well as an assessment of the kidney
injury by ACR measurement should be performed. The eGFR together with the ACR are
used to stage the patient according to the KDIGO heat map from the 2012
guidelines [69]. This staging allows for risk stratification which is crucial to
determine the prognosis of CKD as well as adopt therapy. Another important aspect
of risk stratification is to determine high-risk patients who should be referred
to a nephrologist [90]. A tool that can be used is the kidney failure risk
equation (KFRE) by Tangri et al. [100] which provides a 2- and 5-year
risk of ESKD by using four variables: eGFR, sex, age and albuminuria. The KFRE
was developed in the Canadian population but has been externally validated in 31
multinational cohorts and is therefore applicable worldwide [101]. In the KDIGO
controversies conference the use of risk equations like the KFRE is encouraged to
stratify CKD patients [90]. The National Institute for Health and Care Excellence (NICE) guidelines 2021 on CKD diagnosis and
management even defined a 5-year risk of having ESKD
How often a patient should be screened remains an individual decision, based on the risk stratification and can range from 1 to 10 years [90].
In order to achieve a comprehensive early identification of CKD and lessen the global burden awareness of CKD has to rise. The new KDIGO CKD guidelines, which are to be published in 2023, finally provide a chapter on CKD screening, but further measures must be undertaken on a global and national level [1]. This most importantly includes education of general practitioners and enabling joint efforts of nephrologists, general practitioners as well as doctors from other specialities to identify and treat CKD.
To our knowledge, this is the first manuscript that reports a discussion about eGFR in agreement with the standard of clinical research tools, namely its role as a prognostic biomarker, treatment response predictive biomarker and endpoint biomarker itself. Considered together, these points offer a uniform and complete discussion on the topic and better integrate with future perspectives. Moreover, we also reported, in keeping with the need to continue working on this, the role of eGFR in the awareness of CKD, which is gaining momentum among nephrologists and the public health community. We may contend that eGFR is a strong clinical measure for nephrologists and physicians. It significantly helps to refine risk stratification of patients, to include patients in clinical studies and to assess the response to nephro- and cardioprotective treatments. Moreover, eGFR is a useful and very cheap tool, combined with urine examinations, to screen the general population for kidney disease and, thus, to increase awareness of CKD overall. Future studies are needed to implement the use of eGFR in clinical research and practice.
These should be presented as follows: CA, MP and OB designed the research study. CA, MP, SHK, LH, GLM, GC and OB performed the research. CA, MP, SHK, LH, VC, GLM, GC and OB provided help and advice on specific parts of the article. CA, MP, SHK, GC and OB wrote the manuscript. All authors contributed to editorial changes in the manuscript. All authors read and approved the final manuscript. All authors have participated sufficiently in the work and agreed to be accountable for all aspects of the work.
Not applicable.
Not applicable.
This research received no external funding.
The authors declare no conflict of interest. Michele Provenzano is serving as Guest Editor of this journal. We declare that Michele Provenzano had no involvement in the peer review of this article and has no access to information regarding its peer review. Full responsibility for the editorial process for this article was delegated to Giuseppe Boriani and Alessandro Cataliotti.
Publisher’s Note: IMR Press stays neutral with regard to jurisdictional claims in published maps and institutional affiliations.