- Academic Editor
Background: Atrial fibrillation is the most common tachyarrhythmia, while catheter ablation is an effective therapy for atrial fibrillation. However, pain and nervousness may occur during the procedure. Moreover, a consensus has still not been reached on which is the best kind of analgesic and sedative to use in these procedures. Therefore, we conducted a network meta-analysis to evaluate the efficacy and safety of analgesics and sedatives used in catheter ablation for atrial fibrillation. Methods: We searched PubMed, Cochrane Library, Web of Science, EMBASE, China National Knowledge Infrastructure, and Baidu Wenku document download website for randomized controlled trials from their inception to February 26, 2023. Only studies that made comparisons among analgesics or sedatives and involved patients with atrial fibrillation undergoing radiofrequency catheter ablation were included. The efficacy endpoints were Ramsay sedation scores and visual analog scale scores during the radiofrequency catheter ablation for atrial fibrillation. The safety endpoints were the incidence of respiratory depression, hypotension, nausea, and vomiting. Pairwise comparisons and frequency method analyses were conducted. Results were reported as odds ratio (OR), mean difference (MD), and corresponding 95% confidence intervals (CIs). We assessed the risk bias of the studies in accordance with the Cochrane Handbook for Systematic Reviews of Interventions. Results: Out of the 709 articles initially retrieved, 14 studies, with a total of 1156 participants, were included. In terms of efficacy, patients receiving dexmedetomidine during radiofrequency ablation for atrial fibrillation had higher Ramsay sedation scores than those receiving midazolam plus fentanyl, or its derivatives (MD –0.88, 95% CI [–0.04 to –0.72]). Compared with morphine, dezocine (MD 1.88, 95% CI [1.16 to 2.60]), hydromorphone (MD 4.07, 95% CI [3.56 to 4.58]), butorphanol (MD 3.18, 95% CI [2.38 to 3.96]), and fentanyl or its derivatives (MD 1.57, 95% CI [1.25 to 1.89]) had a better analgesic effect. In terms of safety, propofol (OR 16.46; 95% CI [1.54 to 175.95]) and midazolam plus fentanyl or its derivatives (OR 7.02; 95% CI [1.33 to 36.99]) significantly increased the incidence of respiratory depression compared with dexmedetomidine plus fentanyl or its derivatives. Dexmedetomidine plus fentanyl or its derivatives reduced the incidence of nausea and vomiting compared with fentanyl alone (OR 4.74; 95% CI [1.01 to 22.22]). Propofol was associated with a lower incidence of nausea and vomiting than hydromorphone (OR 0.01; 95% CI [0.00 to 0.59]) and fentanyl or its derivatives (OR 0.01; 95% CI [0.00 to 0.51]). There was no statistically significant difference in the incidence of hypotension between any two strategies. Conclusions: Hydromorphone and butorphanol had better analgesic effects than fentanyl or its derivates. Dexmedetomidine had better sedative effects. In terms of safety, dexmedetomidine, oxymorphone, and butorphanol were superior. It is necessary to explore the regimen that can consider both the effectiveness and safety during radiofrequency catheter ablation for atrial fibrillation (AF). The PROSPERO Registration: This study was registered with PROSPERO, number: CRD42023403661.
Atrial fibrillation (AF) is a common tachyarrhythmia, which increases in incidence each year. Radiofrequency catheter ablation (RFA) is a minimally invasive procedure for the treatment of AF. At present, RFA in patients with AF has become an important treatment method. Randomized controlled trials (RCTs) have shown that RFA for AF has advantages over drug therapy in the treatment of paroxysmal and persistent atrial fibrillation [1]. A catheter is placed into the atrium through venipuncture, and three-dimensional modeling of the atrium is carried out, with the assistance of the CARTO three-dimensional mapping system (Biosense Webster, Inc., Diamond Bar, CA, USA), to search for the substrate causing AF and apply high-frequency current for the ablation of myocardial tissue, to ablate the arrhythmia. However, high-frequency currents will cause some thermal damage to the myocardium during the procedure, and the three-position mapping system requires patients to maintain a certain position for a long time. As a result, patients may be unable to tolerate the pain or move their limbs. This can lead to the displacement of three-dimensional images, thus, affecting the accuracy of the ablation targets, prolonging the operation time, and increasing the possibility of postoperative complications. Therefore, the implementation of a safe and effective sedation and analgesia strategy is crucial to ensure the success of RFA procedures.
The main sedative and analgesic drugs used in RFA for patients with atrial
fibrillation include opioids, benzodiazepines,
We followed the Preferred Reporting Items for Systematic Reviews and Meta-Analyses (PRISMA) guidelines. Additionally, we developed a protocol and registered it on PROSPERO (CRD42023403661).
We performed a systematic review and network meta-analysis. We searched the PubMed, Cochrane Library, Web of Science, EMBASE, China National Knowledge Infrastructure, and Baidu Wenku document download website for randomized controlled trials from the date of their inception to Feb 26, 2023, without language restrictions. To ensure the comprehensiveness of the retrieval, a combination of subject terms and free terms was used for the literature retrieval. All search results were stored using Endnote software (Thomson Corporation, Stanford, CT, USA) for further filtration. We used the following search keywords: “atrial fibrillation”, “auricular fibrillation”, “AF”, “analgesia”, “analgesic”, “sedation”, “sedative”, “radiofrequency ablation”, and “catheter ablation”, which were combined with analgesic and sedative drugs currently used in clinical practice, such as “morphine” and “fentanyl”. We only included RCTs that compared different analgesic or sedative strategies in patients with AF undergoing RFA.
The inclusion criteria were as follows: (1) patients diagnosed with AF undergoing RFA; (2) study design was an RCT to compare different analgesics or sedatives during RFA; (3) study with outcomes of “Ramsay sedation scores (RSS)”, “visual analog scale (VAS)”, “incidence of respiratory depression”, “incidence of hypotension”, or “incidence of nausea and vomiting”; (4) studies with two or multiple arms.
The exclusion criteria were as follows: (1) study types were reviews, observational studies, registry data, ongoing trials without results, case reports, systematic reviews, meta-analysis, animal experimental studies, or duplicate studies; (2) studies without definition of endpoints; (3) unrelated studies; (4) study data could not be obtained.
Our endpoints were the efficacy of an analgesic or sedative, evaluated using RSS
and VAS scores. The RSS was defined: one point: the patient is awake but anxious,
agitated, or restless; two points: the patient is awake but cooperative,
orientated, and tranquil; three points: the patient is drowsy but responsive to
commands; four points: the patient is asleep and with brisk response to glabella
tap or loud auditory stimulus; five points: the patient is asleep with sluggish
response to a stimulus; six points: the patient has no response to noxious
stimuli. Pain was assessed by VAS, using a scale of 0 to 10. The VAS rules were
as follows: higher scores represent more intense pain, a score of 0 represents no
pain, and 10 points represent severe pain. The safety endpoints were the
incidences of respiratory depression, hypotension, nausea, and vomiting during
ablation therapy. The trial defined respiratory depression as SaO
The search results were screened independently by two blinded researchers (LJ and LZ), according to inclusion and exclusion criteria. Disagreements were resolved through discussions and referral to a third coauthor (HG). Two authors independently extracted the following data from the included RCTs: the first author’s name, the publication year, the baseline characteristics, the number of patients in each group, interventions, procedure duration, Ramsay sedation scores, analgesic scores, and occurrence of adverse reactions.
Two authors independently accessed the quality and risk of bias of the included studies using the Cochrane Collaboration Systematic Evaluators manual. We used Review Manager software (Version 5.4, Cochrane, London, United Kingdom) to produce the risk of bias graph. To assess publication bias, we generated funnel graphs using STATA software (Version 17.0, StataCorp, Texas City, TX, USA).
The data were analyzed using STATA software (Version 17.0) based on a frequency model. First, we drew network evidence plots to show direct comparisons. We plotted network contributions to show the contribution of direct comparisons to indirect comparisons. We used “ifplot” in the “network” software package (Thomson Corporation, Stanford, CT, USA) to evaluate inconsistencies. The inconsistency factor (IF) and IF 95% confidence interval (CI) were used to evaluate differences between direct and indirect comparisons. If 95% CI of IF contains 0, the consistency is high. According to the inclusion and exclusion criteria, only RCTs were included in the network meta-analysis, meaning there was no loop formed regarding the efficacy endpoints and the incidence of hypotension, nausea, and vomiting. An inconsistency test was not required to evaluate the results of the direct comparison and indirect comparison. Then, interval plots were drawn. We performed league tables to show the network meta-analysis (NMA) results. To compare each strategy, we used the surface under the cumulative ranking curve (SUCRA) to estimate the probabilities (%) of each treatment, as being the best or other rankings. Finally, we developed correction funnel plots to determine the evidence of the small sample effect and publication bias.
We retrieved 708 articles from the PubMed, Cochrane Library, Web of Science, EMBASE, and China National Knowledge Infrastructure databases, and 1 article from the Baidu Wenku document download website. A total of 104 duplicate articles were excluded. After reading the titles and abstracts, 540 articles were removed, and 51 articles were further screened by reading the full text. Finally, 14 RCTs were included in the NMA, including 13 from the database and 1 from the Baidu Wenku document download website. Fig. 1 illustrates the literature screening process. The 14 studies [2, 3, 4, 5, 6, 7, 8, 9, 10, 11, 12, 13, 14, 15] involved 1156 patients, 9 drugs, and 10 regimens, which were propofol, dexmedetomidine, fentanyl or its derivatives, butorphanol, oxycodone, hydromorphone, dezocine, morphine, dexmedetomidine combined with fentanyl or its derivatives, and midazolam combined with fentanyl or its derivatives. The studies and the patient characteristics are shown in Table 1 (Ref. [2, 3, 4, 5, 6, 7, 8, 9, 10, 11, 12, 13, 14, 15]). All included studies were RCTs. The risk of bias and quality evaluation results for the studies are shown in Fig. 2.
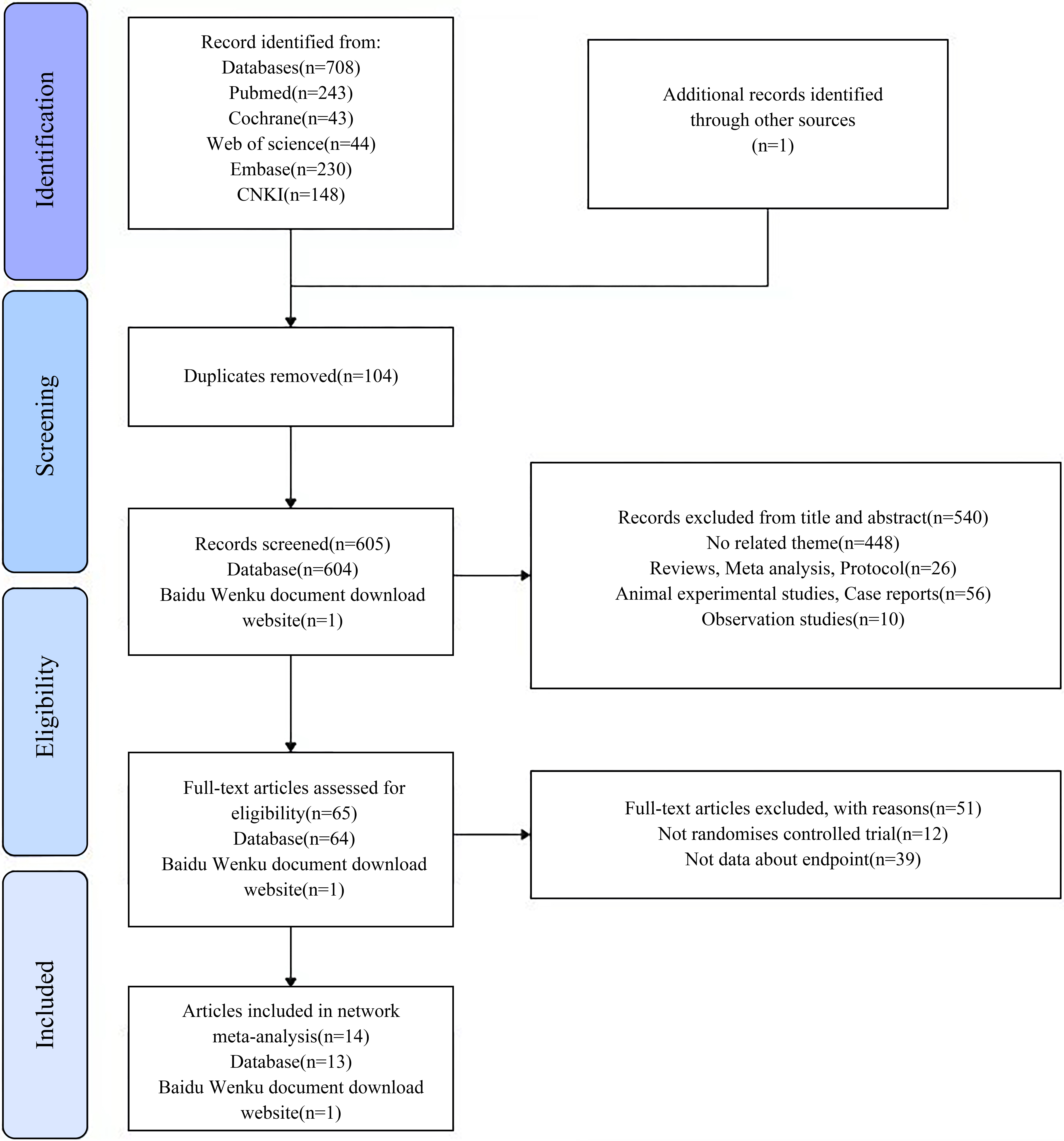
The Preferred Reporting Items for Systematic Reviews and Meta-Analyses (PRISMA) flowchart for this network meta-analysis. CNKI, Chinese National Knowledge Infrastructure.
Study | Intervention | Sample size | Age | Gender (female/male) | Weight (kg) | ASA (I/II/III) | Procedure time (min) | Outcomes reported | Race | Study setting | |||||||
CG | EG | CG | EG | CG | EG | CG | EG | CG | EG | CG | EG | CG | EG | ||||
Servatius H, et al. 2022 [2] | PRO | DEX | 80 | 80 | 64.2 |
65.5 |
23/57 | 28/52 | — | — | 0/66/14 | 0/58/22 | — | — | ② | European | Single-center, single-blind |
Cho JS, et al. 2014 [3] | DEX+F | MD+F | 45 | 45 | 55.2 |
56.3 |
36/9 | 36/9 | 70.9 |
72.9 |
16/29/0 | 8/37/0 | 199.7 |
210.1 |
①②④ | Asian | Sigle-center, single-blind |
Tang RB, et al. 2007 [4] | PRO | MD+F | 60 | 60 | — | — | — | — | — | — | — | — | — | — | ②④ | Asian | Sigle-center, single-blind |
Gu XK, 2020 [5] | F | BT | 40 | 40 | 58.7 |
57.4 |
12/28 | 14/26 | 73.2 |
70.0 |
24/16/0 | 26/14/0 | 160.6 |
159.8 |
①②③④ | Asian | Sigle-center, single-blind |
Chang EQ, et al. 2020 [8] | F | OXY | 50 | 50 | 54.8 |
52.5 |
27/21 | 29/23 | 66.0 |
67.9 |
19/32/0 | 18/31/0 | — | — | ② | Asian | Sigle-center, double-blind |
Ding N, et al. 2018 [9] | F | HMOR | 30 | 30 | 65.3 |
65.4 |
14/16 | 12/18 | 66.5 |
69.7 |
— | — | 120.4 |
126.2 |
①②③④ | Asian | Sigle-center, double-blind |
Ni WJ, 2020 [10] | MD+F | DEX+F | 23 | 25 | 61.6 |
63.2 |
13/10 | 17/8 | 70.8 |
74.5 |
— | — | 151.3 |
142.8 |
①②④ | Asian | Sigle-center |
Yuan SP, et al. 2021 [11] | F | DEX+F | 52 | 43 | 55.3 |
54.3 |
30/22 | 25/18 | — | — | — | — | 213.4 |
217.2 |
①② | Asian | Sigle-center |
Long XF, et al. 2017 [12] | F | DEX+F | 40 | 40 | 58.5 |
59.2 |
25/15 | 26/14 | 60.4 |
62.6 |
21/19/0 | 23/17/0 | 218.1 |
212.4 |
①②④ | Asian | Sigle-center, triple blind |
Yuan JF, et al. 2014 [13] | F | DEX+F | 30 | 30 | 50-65 | 50-65 | — | — | — | — | — | — | 220.0 |
206.0 |
①④ | Asian | Sigle-center |
Chen HY, et al. 2018 [14] | DEX | MD+F | 24 | 24 | 46.0 |
48.0 |
18/6 | 20/4 | — | — | — | — | — | — | ①② | Asian | Sigle-center |
Li KY, et al. 2021 [15] | MOR | F | 40 | 40 | 66.7 |
65.7 |
22/18 | 23/17 | 73.0 |
70.7 |
— | — | 96.9 |
104.0 |
①②③④ | Asian | Sigle-center |
Tan J, et al. 2016 [6] | MOR | DZ | 30 | 60 | 60.1 |
59.3 |
20/10 | 40/20 | 66.0 |
65.1 |
— | — | 252.4 |
202.3 |
③ | Asian | Sigle-center |
Li FZ, et al. 2015 [7] | MOR | DZ | 20 | 25 | 54.6 |
61.0 |
13/7 | 12/13 | — | — | — | — | 258.0 |
194.4 |
③ | Asian | Sigle-center |
CG, control group; EG, experimental group; PRO, propofol; DEX, dexmedetomidine; F, fentanyl or its derivatives; BT, butorphanol; OXY, oxycodone; HMOR, hydromorphone; MOR, morphine; MD, midazolam; DZ, dezocine; ASA, American Society of Aneshesiologists. ①: intraoperative sedation scores; ②: incidence of respiratory depression; ③: intraoperative analgesic scores; ④: incidence of nausea and vomiting.
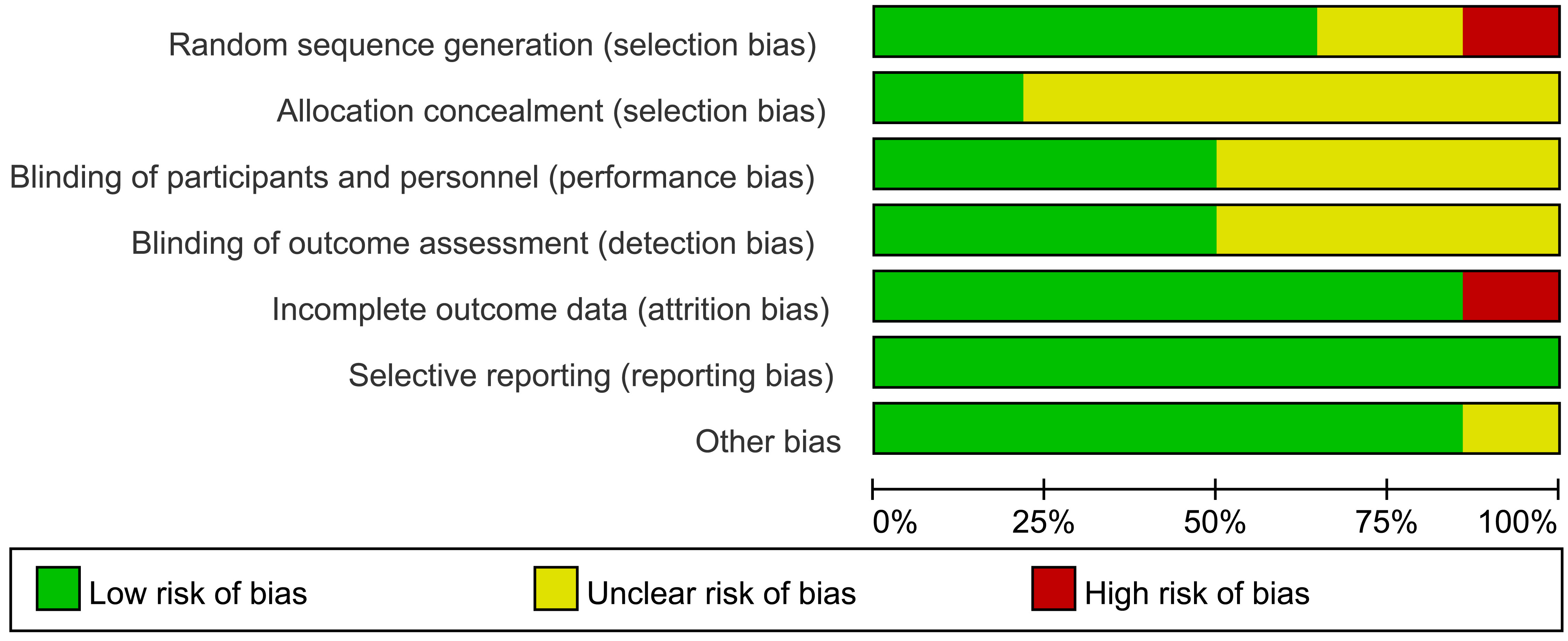
Risk of bias graph.
RSS and VAS scores were primary efficacy outcome indexes. Out of the 14 included
studies, 9 studies [3, 5, 9, 10, 11, 12, 13, 14, 15] reported RSS and involved 7 regimens. Fig. 3a
shows the network plot. Fig. 3b shows the contribution plot for RSS. Paired
comparisons among the seven medication regimens revealed that patients receiving
dexmedetomidine during RFA for AF had higher RSS than those receiving midazolam
plus fentanyl or its derivatives (mean difference [MD] –0.88, 95% CI [–0.04 to
–0.72], p
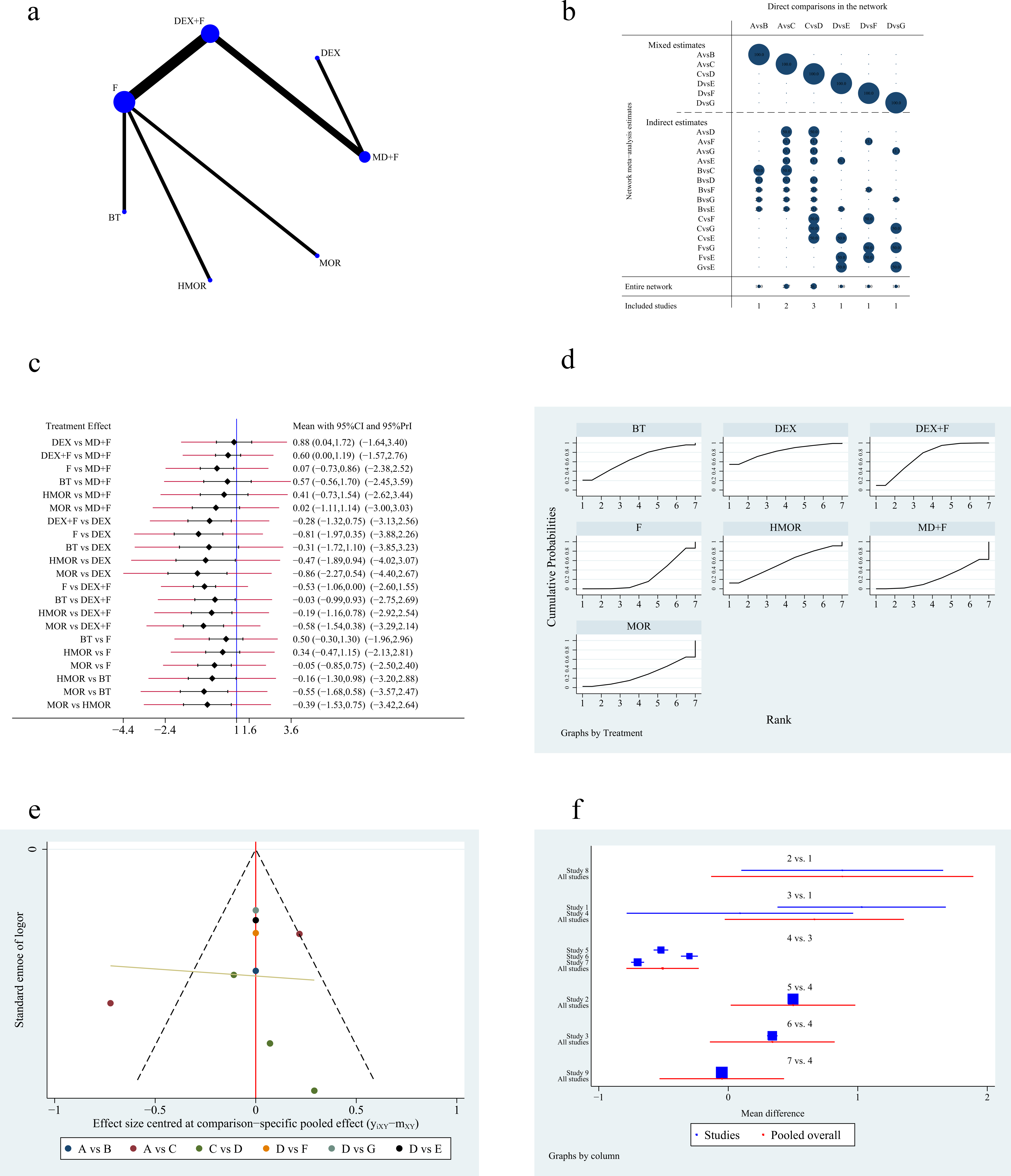
Figures of network meta-analysis of Ramsay sedation scores. (a) Network plot of Ramsay sedation scores. Line thickness represents the number of comparisons between the two arms, while node size represents the sample size of each arm. (b) Contribution plot of Ramsay sedation scores. (c) Prediction intervals map of Ramsay sedation scores. (d) SUCRA plot of Ramsay sedation scores. (e) Funnel plot. (f) Forest plot of Ramsay sedation scores. DEX, dexmedetomidine; F, fentanyl or its derivatives; BT, butorphanol; HMOR, hydromorphone; MOR, morphine; MD, midazolam; CI, confidence interval; PrI, prediction interval; SUCRA, the surface under the cumulative ranking curve. A: midazolam plus fentanyl or its derivatives, B: dexmedetomidine, C: dexmedetomidine plus fentanyl or its derivatives, D: fentanyl or its derivatives, E: butorphanol, F: hydromorphone, G: morphine.
MOR | –0.02 (–1.14, 1.11) | 0.39 (–0.75, 1.53) | 0.05 (–0.75, 0.85) | 0.58 (–0.38, 1.54) | 0.86 (–0.54, 2.27) | 0.55 (–0.58, 1.68) |
0.02 (–1.11, 1.14) | MD+F | 0.41 (–0.73, 1.54) | 0.07 (–0.73, 0.86) | 0.60 (–0.00, 1.19) | 0.88 (0.04, 1.72) | 0.57 (–0.56, 1.70) |
–0.39 (–1.53, 0.75) | –0.41 (–1.54, 0.73) | HMOR | –0.34 (–1.15, 0.47) | 0.19 (–0.78, 1.16) | 0.47 (–0.94, 1.89) | 0.16 (–0.98, 1.30) |
–0.05 (–0.85, 0.75) | –0.07 (–0.86, 0.73) | 0.34 (–0.47, 1.15) | F | 0.53 (–0.00, 1.06) | 0.81 (–0.35, 1.97) | 0.50 (–0.30, 1.30) |
–0.58 (–1.54, 0.38) | –0.60 (–1.19, 0.00) | –0.19 (–1.16, 0.78) | –0.53 (–1.06, 0.00) | DEX+F | 0.28 (–0.75, 1.32) | –0.03 (–0.99, 0.93) |
–0.86 (–2.27, 0.54) | –0.88 (–1.72, –0.04) | –0.47 (–1.89, 0.94) | –0.81 (–1.97, 0.35) | –0.28 (–1.32, 0.75) | DEX | –0.31 (–1.72, 1.10) |
–0.55 (–1.68, 0.58) | –0.57 (–1.70, 0.56) | –0.16 (–1.30, 0.98) | –0.50 (–1.30, 0.30) | 0.03 (–0.93, 0.99) | 0.31 (–1.10, 1.72) | BT |
NMA, network meta-analysis; DEX, dexmedetomidine; F, fentanyl or its derivatives; BT, butorphanol; HMOR, hydromorphone; MOR, morphine; MD, midazolam.
Five studies [5, 6, 7, 9, 15] reported VAS scores involving five regimens. Fig. 4a,b
shows the network plot and contribution plot of the analgesic scores. The NMA
results showed that compared to morphine, dezocine (MD 1.88, 95% CI [1.16 to
2.60], p
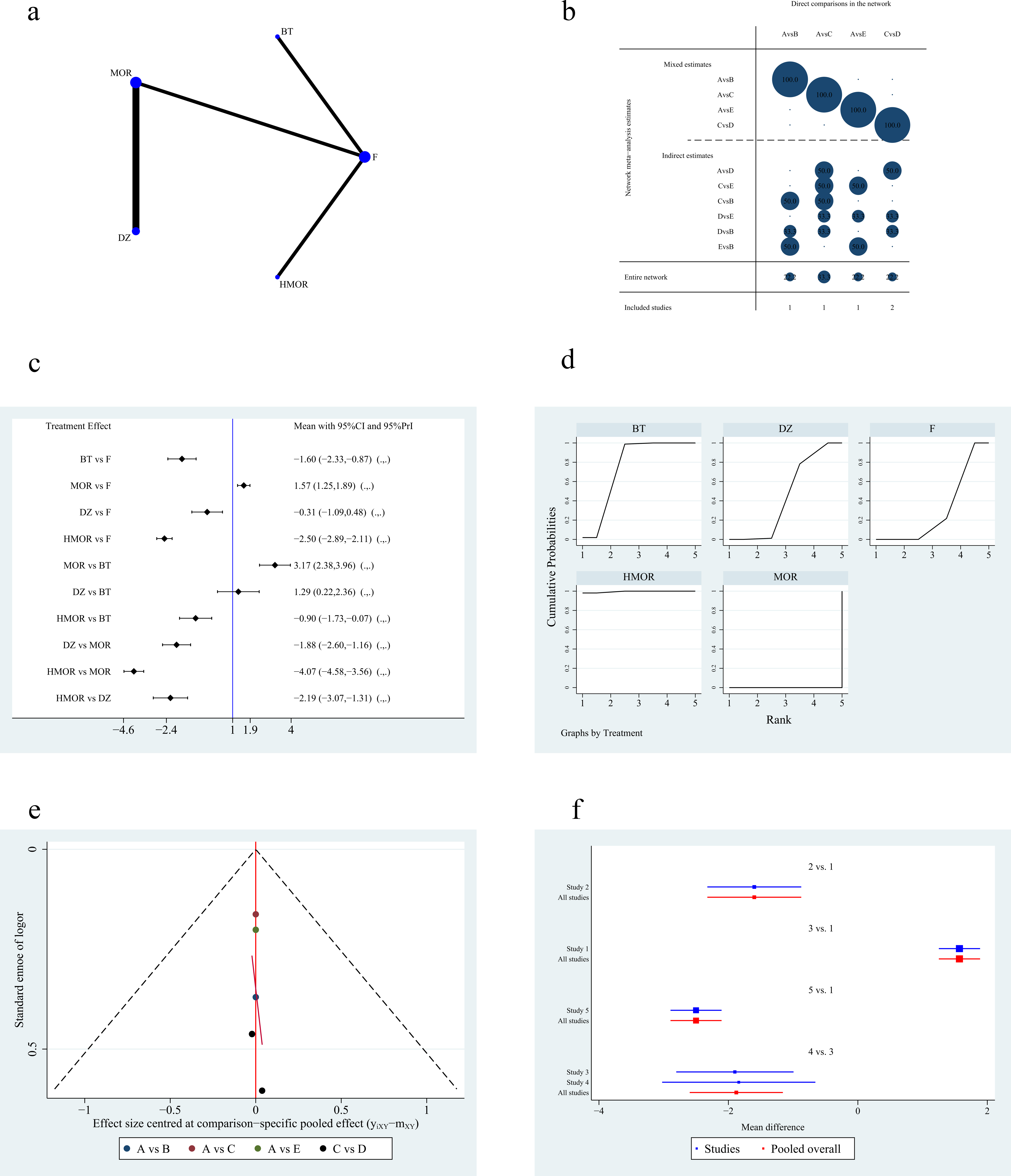
Figures of network meta-analysis of VAS. (a) Network plot of VAS. Line thickness represents the number of comparisons between the two arms, while node size represents the sample size of each arm. (b) Contribution plot of VAS. (c) Prediction intervals map of VAS. (d) SUCRA plot of VAS. (e) Funnel plot. (f) forest plot of VAS. F, fentanyl or its derivatives; BT, butorphanol; HMOR, hydromorphone; MOR, morphine; DZ, dezocine; CI, confidence interval; VAS, visual analog scale; SUCRA, the surface under the cumulative ranking curve; PrI, prediction interval. A: fentanyl or its derivatives, B: butorphanol, C: morphine, D: dezocine, E: hydromorphone.
MOR | –4.07 (–4.58, –3.56) | –1.57 (–1.89, –1.25) | –1.88 (–2.60, –1.16) | –3.17 (–3.96, –2.38) |
4.07 (3.56, 4.58) | HMOR | 2.50 (2.11, 2.89) | 2.19 (1.31, 3.07) | 0.90 (0.07, 1.73) |
1.57 (1.25, 1.89) | –2.50 (–2.89, –2.11) | F | –0.31 (–1.09, 0.48) | –1.60 (–2.33, –0.87) |
1.88 (1.16, 2.60) | –2.19 (–3.07, –1.31) | 0.31 (–0.48, 1.09) | DZ | –1.29 (–2.36, –0.22) |
3.17 (2.38, 3.96) | –0.90 (–1.73, –0.07) | 1.60 (0.87, 2.33) | 1.29 (0.22, 2.36) | BT |
NMA, network meta-analysis; F, fentanyl or its derivatives; BT, butorphanol; HMOR, hydromorphone; MOR, morphine; DZ, dezocine.
The safety outcomes reported were complication rates, mainly consisting of
respiratory depression, hypotension, nausea, and vomiting. For the safety
outcome, 11 studies [2, 3, 4, 5, 8, 9, 10, 11, 12, 14, 15] reported rates of respiratory depression.
Fig. 5a,b shows the network plot and contribution plot of the incidence of
respiratory depression. We conducted inconsistencies tests based on the loop, and
the results showed that the 95% CI of IF contained 0, meaning that there was no
obvious inconsistency; therefore, we used the consistency model for NMA (Fig. 5c). Dexmedetomidine combined with fentanyl or its derivatives significantly
reduced the incidence of respiratory depression compared with propofol (odds
ratio [OR] 16.46; 95% CI [1.54 to 175.95], p
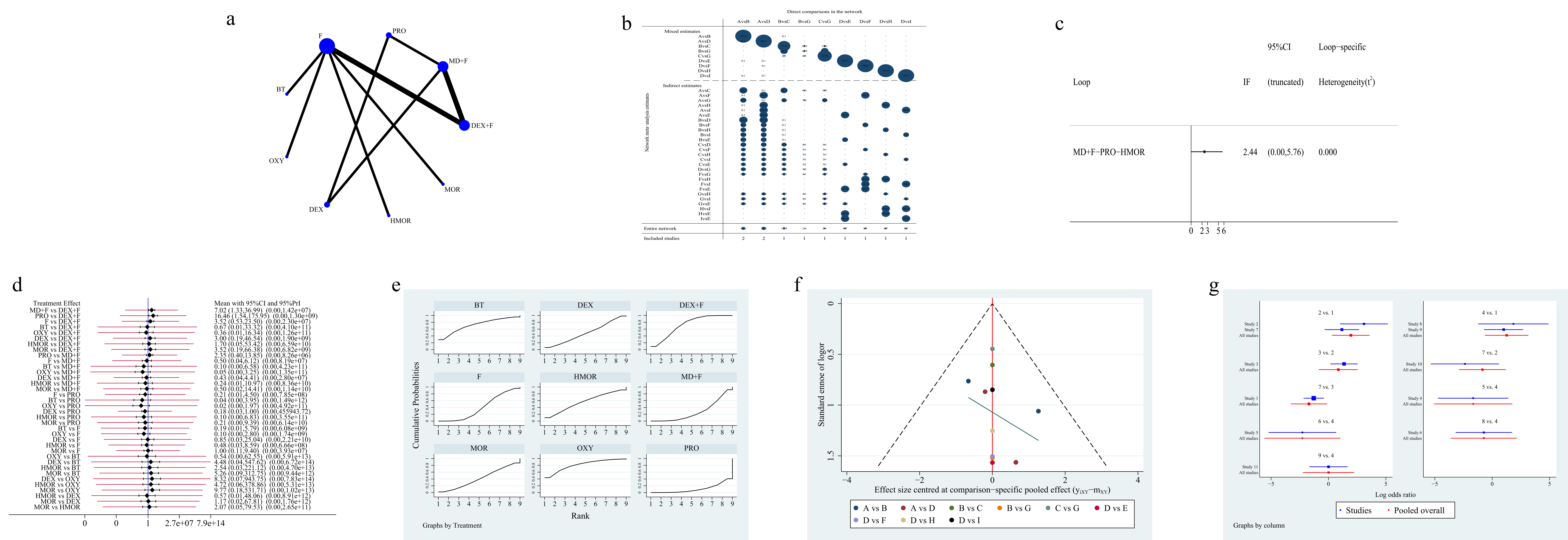
Figures of network meta-analysis of respiratory despression. (a) Network plot of respiratory depression. Line thickness represents the number of comparisons between the two arms, while node size represents the sample size of each arm. (b) Contribution plot of respiratory depression. (c) Inconsistency test plot of respiratory depression. (d) Prediction intervals map of respiratory depression. (e) SUCRA plot of respiratory depression. (f) Funnel plot. (g) Forest plot of respiratory depression. F, fentanyl or its derivatives; BT, butorphanol; HMOR, hydromorphone; MOR, morphine; OXY, oxycodone; PRO, propofol; DEX, dexmedetomidine; MD, midazolam; SUCRA, the surface under the cumulative ranking curve; CI, confidence interval; PrI, prediction interval. A: dexmedetomidine plus fentanyl or its derivatives, B: midazolam plus fentanyl or its derivatives, C: propofol, D: fentanyl or its derivatives, E: butorphanol, F: oxycodone, G: dexmedetomidine, H: hydromorphone, I: morphine.
PRO | 0.02 (0.00, 1.97) | 0.21 (0.00, 9.39) | 0.43 (0.07, 2.52) | 0.10 (0.00, 6.83) | 0.21 (0.01, 4.50) | 0.06 (0.01, 0.65) | 0.18 (0.03, 1.00) | 0.04 (0.00, 3.95) |
45.73 (0.51, 4109.80) | OXY | 9.77 (0.18, 531.71) | 19.49 (0.31, 1233.80) | 4.72 (0.06, 378.86) | 9.77 (0.36, 267.37) | 2.78 (0.06, 126.04) | 8.32 (0.07, 943.75) | 1.86 (0.02, 216.01) |
4.68 (0.11, 205.56) | 0.10 (0.00, 5.57) | MOR | 1.99 (0.07, 57.31) | 0.48 (0.01, 18.54) | 1.00 (0.11, 9.40) | 0.28 (0.02, 5.36) | 0.85 (0.01, 49.19) | 0.19 (0.00, 11.30) |
2.35 (0.40, 13.85) | 0.05 (0.00, 3.25) | 0.50 (0.02, 14.41) | MD+F | 0.24 (0.01, 10.97) | 0.50 (0.04, 6.12) | 0.14 (0.03, 0.75) | 0.43 (0.04, 4.41) | 0.10 (0.00, 6.58) |
9.69 (0.15, 641.08) | 0.21(0.00, 17.02) | 2.07 (0.05, 79.53) | 4.13 (0.09, 187.13) | HMOR | 2.07 (0.12, 36.84) | 0.59 (0.02, 18.51) | 1.76 (0.02, 149.60) | 0.39 (0.00, 34.30) |
4.68 (0.22, 98.54) | 0.10 (0.00, 2.80) | 1.00 (0.11, 9.40) | 1.99 (0.16, 24.32) | 0.48 (0.03, 8.59) | F | 0.28 (0.04, 1.90) | 0.85 (0.03, 25.04) | 0.19 (0.01, 5.79) |
16.46 (1.54, 175.95) | 0.36 (0.01, 16.34) | 3.52 (0.19, 66.38) | 7.02 (1.33, 36.99) | 1.70 (0.05, 53.42) | 3.52 (0.53, 23.50) | DEX+F | 3.00 (0.19, 46.54) | 0.67 (0.01, 33.32) |
5.49 (1.00, 30.26) | 0.12 (0.00, 13.62) | 1.17 (0.02, 67.81) | 2.34 (0.23, 24.19) | 0.57 (0.01, 48.06) | 1.17 (0.04, 34.51) | 0.33 (0.02, 5.18) | DEX | 0.22 (0.00, 27.29) |
24.61 (0.25, 2393.88) | 0.54 (0.00, 62.55) | 5.26 (0.09, 312.75) | 10.49 (0.15, 723.40) | 2.54 (0.03, 221.12) | 5.26 (0.17, 160.11) | 1.49 (0.03, 74.44) | 4.48 (0.04, 547.62) | BT |
NMA, network meta-analysis; F, fentanyl or its derivatives; BT, butorphanol; HMOR, hydromorphone; MOR, morphine; OXY, oxycodone; PRO, propofol; DEX, dexmedetomidine; MD, midazolam.
Four studies [3, 4, 10, 14] reported the incidence of hypotension in RFA (Fig. 6a,b). Since there was no loop structure in the network plot, no inconsistency
check was required. The NMA results (Table 5) illustrated that compared with
dexmedetomidine, propofol (OR 27.27; 95% CI [0.05 to 15,721.14]), midazolam plus
fentanyl or its derivatives (OR 7.66; 95% CI [0.07 to 845.55]), dexmedetomidine
plus fentanyl or its derivatives (OR 15.67; 95% CI [0.04 to 6089.58]) probably
had a higher occurrence of hypotension, although none of these results were
statistically different (p
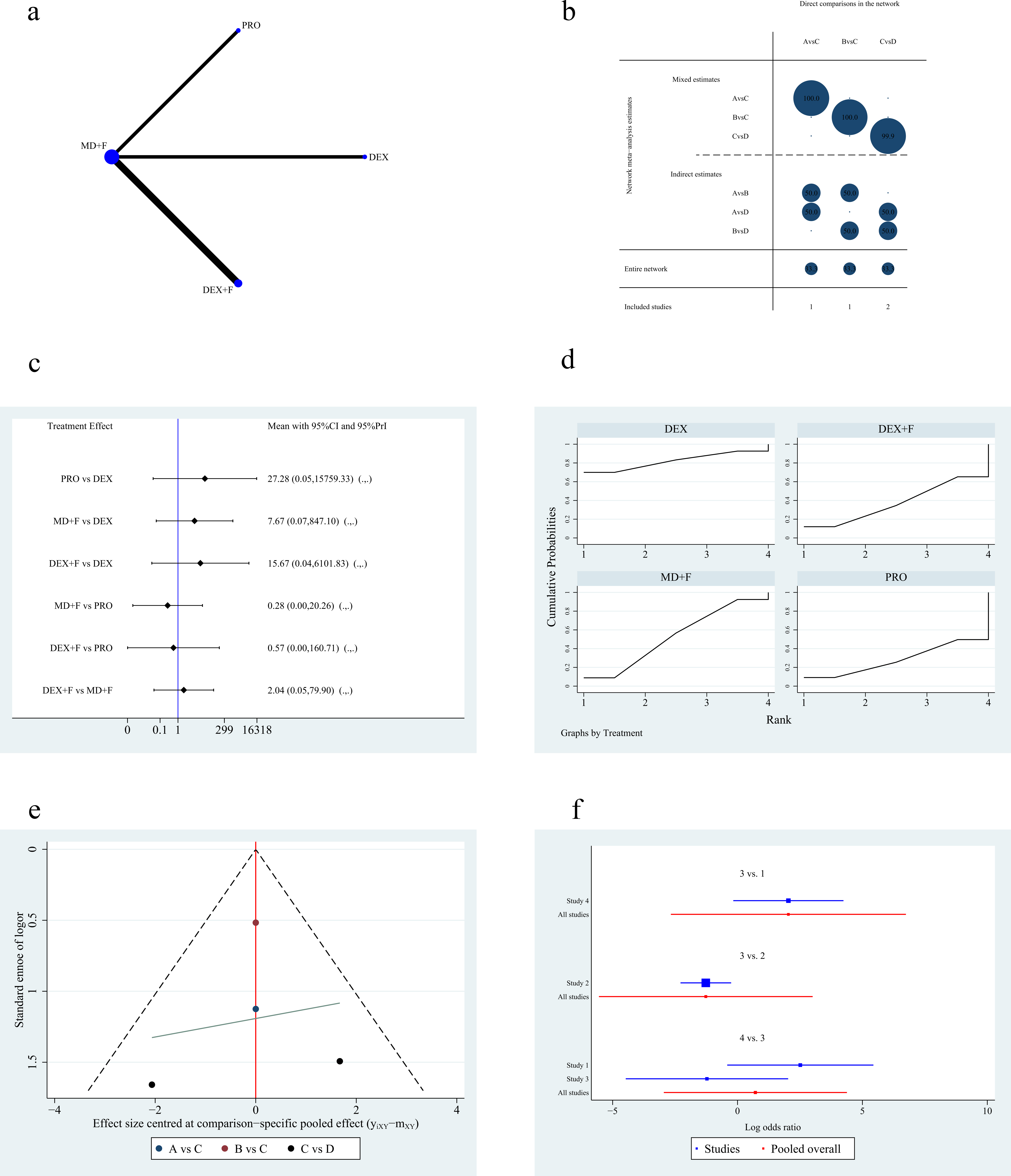
Figures of network meta-analysis of incidence of hypotension. (a) Network plot of incidence of hypotension. Line thickness represents the number of comparisons between the two arms, while node size represents the sample size of each arm. (b) Contribution plot of incidence of hypotension. (c) Prediction intervals map of incidence of hypotension. (d) SUCRA plot of incidence of hypotension. (e) Funnel plot. (f) Forest plot of incidence of hypotension. PRO, propofol; F, fentanyl or its derivatives; MD, midazolam; DEX, dexmedetomidine; SUCRA, the surface under the cumulative ranking curve; CI, confidence interval; PrI, prediction interval. A: dexmedetomidine, B: propofol, C: midazolam plus fentanyl or its derivatives, D: dexmedetomidine plus fentanyl or its derivatives.
PRO | 0.28 (0.00, 20.24) | 0.57 (0.00, 160.50) | 0.04 (0.00, 21.15) |
3.56 (0.05, 256.21) | MD+F | 2.04 (0.05, 79.85) | 0.13 (0.00, 14.40) |
1.74 (0.01, 486.14) | 0.49 (0.01, 19.11) | DEX+F | 0.06 (0.00, 24.81) |
27.27 (0.05, 15721.14) | 7.66 (0.07, 845.55) | 15.67 (0.04, 6089.58) | DEX |
NMA, network meta-analysis; PRO, propofol; F, fentanyl or its derivatives; MD, midazolam; DEX, dexmedetomidine.
Eight articles [3, 4, 5, 9, 10, 12, 13, 15] reported the incidence of nausea and
vomiting from seven strategies. Fig. 7a,b shows the network plot and contribution
plot of the incidence of nausea and vomiting. Since there was no loop structure
in the network plot, no inconsistency check was required. The NMA results showed
that dexmedetomidine plus fentanyl or its derivatives reduced the incidence of
nausea and vomiting compared with fentanyl alone (OR 4.74; 95% CI [1.01 to
22.22], p
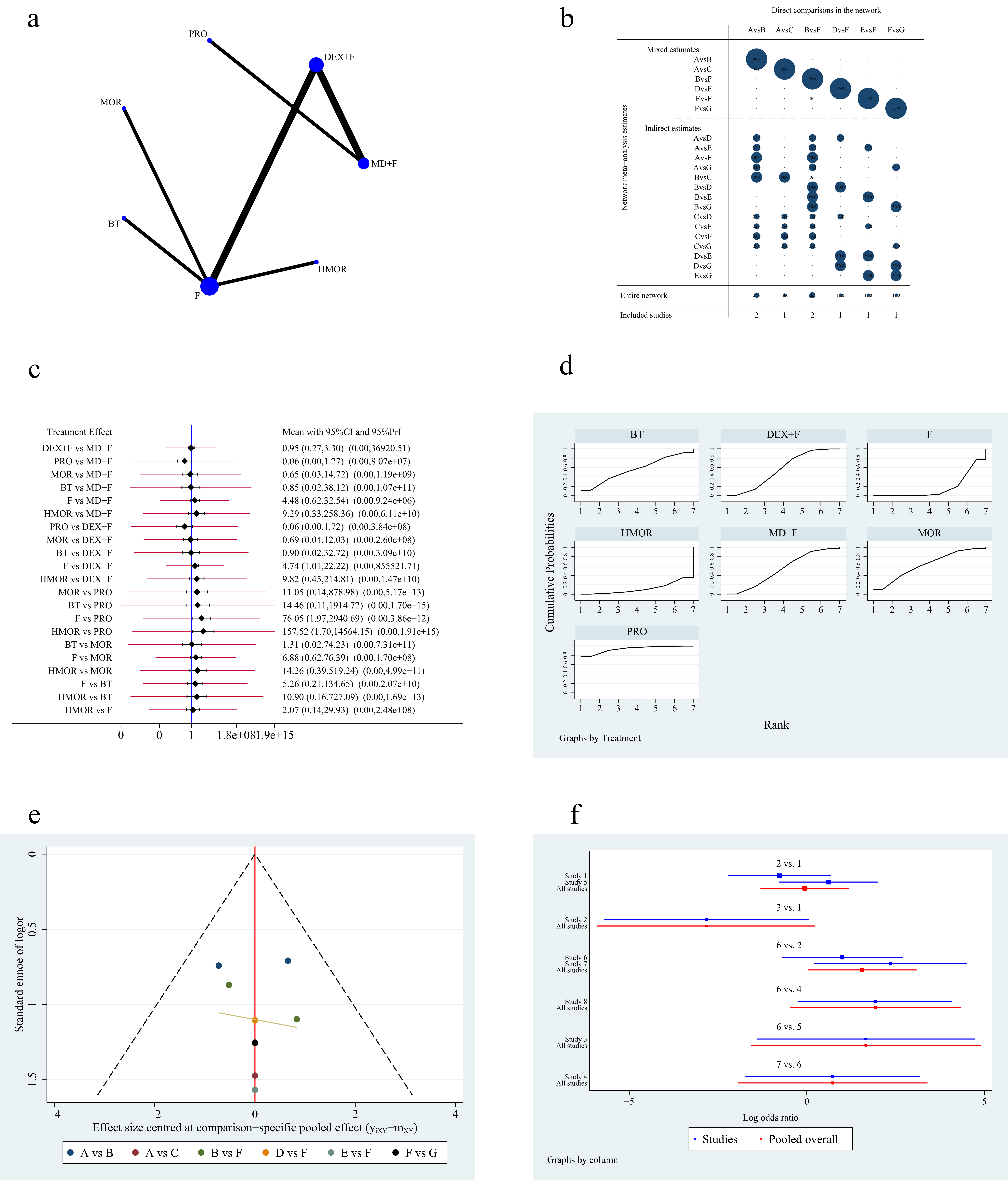
Figures of network meta-analysis of incidence of nausea and vomiting. (a) Network plot of incidence of nausea and vomiting. Line thickness represents the number of comparisons between the two arms, while node size represents the sample size of each arm. (b) Contribution plot of incidence of nausea and vomiting. (c) Prediction intervals map of incidence of nausea and vomiting. (d) SUCRA plot of incidence of nausea and vomiting. (e) Funnel plot. (f) Forest plot of incidence of nausea and vomiting. MD, midazolam; F, fentanyl or its derivatives; DEX, dexmedetomidine; PRO, propofol; MOR, morphine; BT, butorphanol; HMOR, hydromorphone; CI, confidence interval; PrI, prediction interval; SUCRA, the surface under the cumulative ranking curve. A: midazolam plus fentanyl or its derivatives, B: dexmedetomidine plus fentanyl or its derivatives, C: propofol, D: morphine, E: butorphanol, F: fentanyl or its derivatives, G: hydromorphone.
PRO | 11.05 (0.14, 878.91) | 16.96 (0.79, 365.73) | 157.52 (1.70, 14563.17) | 76.05 (1.97, 2940.51) | 16.04 (0.58, 441.39) | 14.46 (0.11, 1914.52) |
0.09 (0.00, 7.20) | MOR | 1.54 (0.07, 34.69) | 14.26 (0.39, 519.21) | 6.88 (0.62, 76.39) | 1.45 (0.08, 25.33) | 1.31 (0.02, 74.22) |
0.06 (0.00, 1.27) | 0.65 (0.03, 14.72) | MD+F | 9.29 (0.33, 258.34) | 4.48 (0.62, 32.54) | 0.95 (0.27, 3.30) | 0.85 (0.02, 38.11) |
0.01 (0.00, 0.59) | 0.07 (0.00, 2.55) | 0.11 (0.00, 3.00) | HMOR | 0.48 (0.03, 6.97) | 0.10 (0.00, 2.23) | 0.09 (0.00, 6.12) |
0.01 (0.00, 0.51) | 0.15 (0.01, 1.61) | 0.22 (0.03, 1.62) | 2.07 (0.14, 29.93) | F | 0.21 (0.05, 0.99) | 0.19 (0.01, 4.87) |
0.06 (0.00, 1.72) | 0.69 (0.04, 12.03) | 1.06 (0.30, 3.69) | 9.82 (0.45, 214.80) | 4.74 (1.01, 22.22) | DEX+F | 0.90 (0.02, 32.72) |
0.07 (0.00, 9.16) | 0.76 (0.01, 43.35) | 1.17 (0.03, 52.46) | 10.90 (0.16, 727.04) | 5.26 (0.21, 134.64) | 1.11 (0.03, 40.25) | BT |
NMA, network meta-analysis; MD, midazolam; F, fentanyl or its derivatives; DEX, dexmedetomidine; PRO, propofol; MOR, morphine; BT, butorphanol; HMOR, hydromorphone.
RFA is an effective treatment strategy for AF, and its success is related to its analgesic and sedative effects. However, analgesic and sedative drugs often lead to adverse reactions. Therefore, selecting an effective and safe drug regimen is crucial. Thus, we conducted this network meta-analysis to compare the efficacy and safety of various analgesic and sedative strategies during RFA for AF. We identified 14 studies, included in NMA; however, all the outcome indicators were only reported in a few studies. Our analyses confirmed several findings: For the analgesic effect, hydromorphone and butorphanol were more prominent, and both dezocine and fentanyl or its derivatives provided better analgesia effects than morphine. For the sedative effect, dexmedetomidine had a higher sedation score compared to midazolam plus fentanyl or its derivatives. In terms of safety endpoints, compared with dexmedetomidine plus fentanyl or its derivatives, propofol and midazolam plus fentanyl or its derivatives were associated with an increased incidence of respiratory depression, while propofol had a lower incidence of nausea and vomiting than hydromorphone and fentanyl or its derivatives. Dexmedetomidine plus fentanyl or its derivatives leads to a lower incidence of nausea and vomiting than fentanyl or its derivatives. There was no statistically significant difference in the incidence of hypotension among these various regimens during RFA for AF.
Opioids, which mainly act on opiate µ,
Dexmedetomidine is a novel
Midazolam is the main benzodiazepine used in RFA for AF. Midazolam works
primarily by increasing the frequency of the chloride channel openings to enhance
the action of the inhibitory neurotransmitter
Propofol, an anesthetic drug that does not have analgesic effects, is used for deep sedation during RFA for AF. Propofol combined with midazolam provides good efficacy and safety in electrical cardioversion [36]. However, propofol presents a risk of dose-related respiratory depression and excessive sedation. NMA showed that propofol deep sedation had lower rates of respiratory depression and hypotension in RFA, yet higher rates of nausea and vomiting. There were only two studies related to propofol, thus, the effectiveness and safety of propofol in RFA for AF need to be verified.
Our study has a few potential limitations. First, we conducted integrated analyses of fentanyl and its derivatives, ignoring the differences and connections between fentanyl, sufentanil, alfentanil, and remifentanil, which may have weakened the credibility of the results. Second, most studies did not report using the blind method and allocation hiding in detail, and some studies did not clarify whether the allocation was random. Third, there were fewer than three studies that included propofol, hydromorphone, oxycodone, and dezocine, and the funnel plot suggested that publication bias might have affected the analysis of these results. Fourth, there was no statistical difference between most indirect comparisons, meaning that the results should be interpreted with caution. Therefore, there is still a need for more high-quality randomized controlled clinical trials to validate these results. Finally, the research populations included in this network meta-analysis were mostly Asian. There are differences in drug response and metabolism among the different ethnic populations, which could affect the interpretation of the network meta-analysis results. Notwithstanding these limitations, the findings from this NMA represent the most current comprehensive available database to guide the use of analgesic and sedative drugs during RFA for AF.
We compared the efficacy and safety of ten analgesic or sedative regimens during RFA for AF. In terms of efficacy, hydromorphone, butorphanol, and dezocine had better analgesic effects than fentanyl. Dexmedetomidine had better sedative effects. In terms of safety, dexmedetomidine, oxymorphone, and butorphanol had the best scores. It is necessary to explore the regimen that can consider both effectiveness and safety during radiofrequency ablation for atrial fibrillation.
The datasets used and/or analyzed during the current study are available from the corresponding author on reasonable request.
LJ and HG designed and monitored the whole analysis. LJ and LZ contributed to the study selection. LJ and LZ contributed to data extraction. FL provided the methodological support. LJ, FL, and HG contributed to the data analysis and paper writing. HG provided the project fund. HG was responsible for the data review. All authors provided critical review and approved this manuscript. All authors read and approved the final manuscript. All authors have participated sufficiently in the work and agreed to be accountable for all aspects of the work.
Not applicable.
Many thanks to everyone who contributed to this work.
This work was supported by the National Nature Science Foundation of China (No.82104844).
The authors declare no conflict of interest.
Publisher’s Note: IMR Press stays neutral with regard to jurisdictional claims in published maps and institutional affiliations.