- Academic Editor
Background: PCSK9 MaB (Proprotein Convertase Subtilisin/Kexin Type 9
Inhibitor) may reduce the occurrence of major adverse cardiovascular events
(MACEs) in patients diagnosed with acute coronary syndrome (ACS). In this
meta-analysis, we conducted a thorough compilation of evidence from established
clinical studies to evaluate PCSK9 MaB’s capacity to control blood lipid levels
and prevent MACEs in ACS patients. Methods: We conducted searches on
Pubmed, Embase, the Cochrane Library, and Web of Science to identify relevant
articles. Data from ACS patients were extracted using a standardized format for
aggregating data. We calculated the risk ratio (RR) for MACE and assessed changes
in blood lipid parameters. All statistical analyses were performed using RevMan.
Results: 11 articles representing 5 trials were included in our
systematic review and meta-analysis. When compared to a placebo, PCSK9 MaB
significantly reduced the risk of MACEs (I
Acute coronary syndrome (ACS) encompasses unstable angina, non-ST-segment elevation myocardial infarction, and ST-segment elevation myocardial infarction [1]. Currently, ACS is highly prevalent among old people, with approximately 85% of ACS-related fatalities occurring in individuals aged 65 or older. It is worth noting that approximately 64% of ACS cases are attributed to the rupture of lipid plaques triggered by inflammation, followed by the formation of platelet-rich thrombosis [2]. In 2023, the European Society of Cardiology (ESC) recommended the combination of high-dose statins and ezetimibe as the standard approach to reducing blood lipid levels in ACS patients [3]. Patients with ACS exhibit a spectrum of clinical symptoms, ranging from mild manifestations like general fatigue to severe symptoms such as breathlessness and, in some cases, intense chest pain, which can pose a life-threatening risk in a short time frame. The pathophysiological changes in coronary syndrome share a common pattern: the transformation of coronary atherosclerotic plaques from a stable state to an unstable one, eventually culminating in plaque rupture and thrombosis [4]. Extensive clinical experience has demonstrated that the perturbation of blood lipid levels [5, 6] further exacerbates plaque instability, increasing the cardiac burden and progressively impeding vascular functions due to delayed blood flow metabolism and blockage of ion transport channels.
Proprotein convertase subtilisin/kexin type 9 inhibitor (PCSK9 MaB), belongs to a class of compounds designed to inhibit PCSK9, the ninth member of the Kexin-like proconvertase subtilisin family [7]. According to the clinical consensus statement endorsed by the Association for Acute Cardiovascular Care (ACVC) in collaboration with the European Association of Preventive Cardiology (EAPC) and the European Society of Cardiology (ESC) Working Group on Cardiovascular Pharmacotherapy, the use of PCSK9 MaB to treat ACS patients is both safe and feasible. This treatment approach aids in plaque stabilization and the prevention of plaque rupture [8].
The current lipid-lowering guidelines from the American College of Cardiology and the American Heart Association recommend adopting intermittent lipid-lowering therapy when an individual’s primary blood lipid levels consistently reach a safe target, such as maintaining low-density lipoprotein cholesterol (LDL-C) at 70 mg/dL through optimized treatment. This approach is especially relevant for patients at a very high risk of experiencing major adverse cardiovascular events (MACEs) [9]. Unsteady lipid-lowering therapy involves clinical treatment in a state of disturbance from external factors, with some interference left or removed, rather than returning to the original state. PCSK9 MaB is a suitable treatment option for these conditions. Such a recommendation is partially grounded in evidence from large clinical trials [8] demonstrating the clinical benefits of this therapy. Numerous prior experiments have confirmed that PCSK9 MaB effectively lowers blood lipid levels in 73.6% of ACS patients [10]. PCSK9 MaB reduces LDL-C, whether administered as monotherapy or in combination with statins. An added benefit of PCSK9 MaB is a 20% to 25% reduction in lipoprotein(a) concentrations [11]. However, approximately one-third of patients receiving PCSK9 MaB exhibit inadequate inhibition of coronary plaque development [12], leaving some patients vulnerable to adverse thrombotic events. The Further Cardiovascular Outcomes Research With PCSK9 monoclonal antibody (FOURIER) trial investigated patients with stable atherosclerotic cardiovascular disease and a previous myocardial infarction (MI). It found that PCSK9 MaB, particularly evolocumab, reduced MACEs in individuals with recent MIs (within 2 years) but did not yield the same effects in those with more remote MIs (beyond 2 years) [11]. As a result, PCSK9 MaB treatment should be considered for all ACS patients with dyslipidemia that remains uncontrolled despite statin therapy. While the effects of PCSK9 MaB have been demonstrated in some studies, it’s worth noting that this treatment can be relatively expensive and may raise concerns about potential MACEs, and its short-term clinical efficacy remains uncertain [13].
Statins have been a longstanding and well-established treatment in clinical practice, known for their clear lipid-lowering effects [3, 14]. In contrast, PCSK9 MaB is relatively new to clinical practice, and its efficacy requires validation through extensive data analysis. Consequently, a meta-analysis was undertaken, encompassing published randomized controlled trials (RCTs) and non-RCTs, to comprehensively assess the efficacy and safety of PCSK9 MaB for ACS.
This study adhered to the Preferred Reporting Items for Systematic reviews and Meta-Analyses (PRISMA) statement and was pre-registered on the PROSPERO platform with the registration number #CRD 42022372809 [15].
We conducted a thorough search of pertinent publications in the PUBMED, EMBASE, Cochrane Library, and Web of Science databases, encompassing the entire period from the inception of these databases up to October 2022. Our search terms were centered around “acute coronary syndrome” and “PCSK9 MaB”. The detailed search strategies and outcomes can be found in Supplementary Table 1.
No language restrictions were imposed during the search. We sought and identified all published studies, including both RCTs and non-RCTs that compared the effectiveness of PCSK9 MaB therapy with conventional or standard therapy for ACS patients. The precise keywords and subject headings employed in our search strategies can be found in Supplementary Table 1.
The inclusion criteria were outlined as follows: (1) Participants were adults, aged 18 years or older. (2) Participants had a history of any type of coronary syndrome, and the diagnostic criteria were explicitly defined in the article. (3) Participants had no mental disorders. (4) Eligible studies were either RCTs or non-RCTs (cohort studies) with independently extractable data. (5) The study population consisted of ACS patients who received PCSK9 MaB. (6) The primary endpoints of the studies encompassed blood lipid levels or clinical outcomes. Clinical ischemic outcomes were characterized as MACEs, which encompassed all-cause mortality, myocardial infarction, stroke, target vessel revascularization, or stent thrombosis.
The following studies were excluded based on the following criteria: (1) those that did not meet the inclusion criteria; (2) animal studies, case reports, conference papers, review articles, and abstracts; (3) studies lacking sufficient data; (4) studies for which access to the original articles and duplicate publications was not feasible; and (5) protocols or studies exclusively focused on genotype-guided strategies.
Two independent investigators conducted a meticulous review of all studies and collected pertinent data using a standardized table. In the event of any discrepancies in data extraction, a third reviewer was engaged in discussions and decision-making. The characteristics of each study were independently extracted, encompassing details such as study design, study population, ethnic background, patient count, average age, treatment approach, follow-up duration, and primary and secondary outcome measures. To assess the methodological quality of the included RCT studies, the Cochrane Collaboration Bias Assessment Tool (ROB 1.0), integrated into Review Manager (version 5.4.1, Northern Europe), was employed.
We employed a random- or fixed-effects model to pool the data. The occurrence of
MACEs or adverse events was compared between the genotype guidance group and the
conventional group. The endpoint outcome was expressed as the risk ratio (RR)
with a corresponding 95% confidence interval (CI). Simultaneously, the level of
heterogeneity was quantified using the I
The reliability of the meta-analysis results was assessed by observing whether the pooled estimates remained consistent (based on the p-value). Statistical analyses were carried out using the Review Manager software (Review Manager (RevMan) [Computer program]. Version 5.4. The Cochrane Collaboration, 2020). All p-values were two-sided, and a p-value less than 0.05 was considered statistically significant.
Overall, this meta-analysis incorporated a total of 11 articles from 5 RCTs, with 7 of these articles pertaining to the ODISSEY trial, as depicted in Fig. 1. No publication bias tests were conducted for any of the outcome measures, given that the number of included studies was fewer than 10.
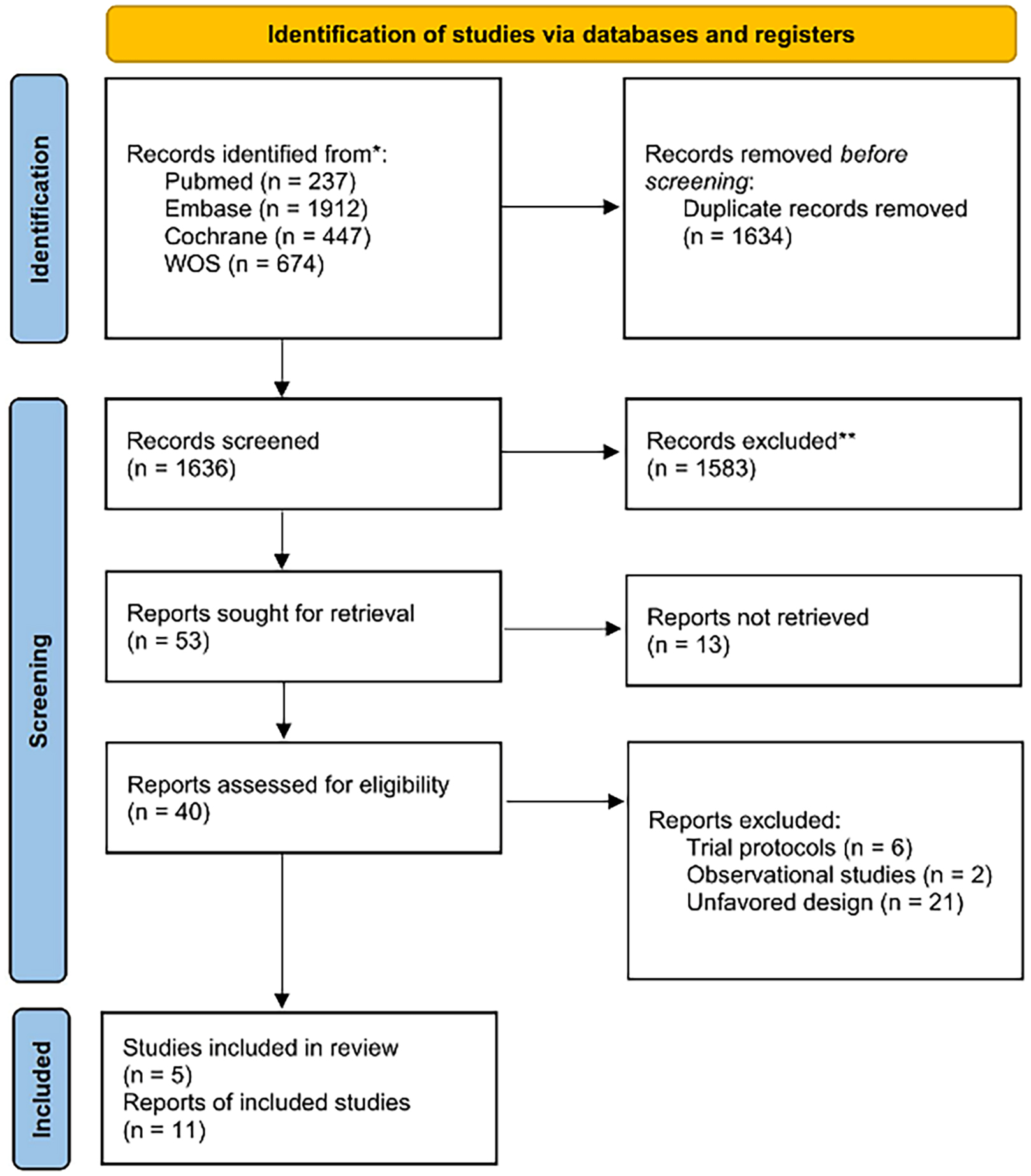
Preferred Reporting Items for Systematic reviews and Meta-Analyses (PRISMA) Flowchart.
The patients had a mean age of 59.38 years, with a standard deviation of 10.6. Among the patients, 14,666 were male (74.6%), while 4983 were female (25.4%). The median follow-up duration ranged from 1.5 to 2.8 years. The PCSK9 MaB group included 9826 patients, and the conventional or standard group included 9823 patients. In the RCTs, no significant disparities in baseline characteristics were detected between the treatment and control groups, as indicated in Table 1 [4, 16, 17, 18, 19, 20, 21, 22, 23, 24, 25].
Author | Year | Region | Population | Age | Gender | PCSK9 MaB | Comparators | |
(mean, SD) | (female, male) | |||||||
Schwartz [16] | 2018 | Multiple | 18,924 | 58.55, 9.35 | 4762, 14,162 | Alirocumab, 70–150 mg, SC, biweekly | Placebo, SC, biweekly | ODISSEY Trial |
Steg [17] | 2019 | Multiple | 18,924 | 58.65, 9.64 | 4762, 14,162 | Alirocumab, 70–150 mg, SC, biweekly | Placebo, SC, biweekly | |
Goodman [18] | 2019 | Multiple | 18,924 | 58.35, 10.34 | 4762, 14,162 | Alirocumab, 70–150 mg, SC, biweekly | Placebo, SC, biweekly | |
Damask [19] | 2020 | Multiple | 11,953 | 58.6, 9.3 | 3039, 8914 | Alirocumab, 70–150 mg, SC, biweekly | Placebo, SC, biweekly | |
Hagström [20] | 2022 | Multiple | 18,924 | 58.28, 10.18 | 4762, 14,162 | Alirocumab, 70–150 mg, SC, biweekly | Placebo, SC, biweekly | |
Schwartz [21] | 2021 | Multiple | 18,924 | 58.19, 9.73 | 4762, 14,162 | Alirocumab, 70–150 mg, SC, biweekly | Placebo, SC, biweekly | |
Schwartz [22] | 2021 | Multiple | 18,490 | 58.18, 9.64 | 4623, 13,867 | Alirocumab, 70–150 mg, SC, biweekly | Placebo, SC, biweekly | |
Koskinas [23] | 2019 | Switzerland | 308 | 60.75, 11.36 | 57, 251 | Evolocumab, 40 mg, everyday | Placebo, 40 mg, everyday | |
Ako [4] | 2019 | Japan | 182 | 61.16, 10.90 | 36, 146 | Alirocumab, 70–150 mg, SC, biweekly | Standard of care | |
Li [24] | 2021 | China | 99 | 59.69, 10.33 | 35, 64 | Evolocumab, 140 mg, SC, biweekly | Placebo, SC, biweekly | |
& Statins, 10 mg, SC, every night | & Statins, 10 mg, SC, every night | |||||||
Hao [25] | 2022 | China | 136 | 62.21, 11.84 | 93, 43 | Evolocumab, 140 mg, SC, biweekly | Placebo, SC, biweekly |
Abbreviation: PCSK9 MaB, proprotein convertase subtilisin/kexin type 9
inhibitor; SD, standard deviation; SC, subcutaneous injection. “Multi” means
“multi-regional”.
Notes:
1. Schwartz, Hagström, Steg, Goodman, Damask published 7
articles concerning the results of different populations based on ODISSEY.
2. Standard of care: It was defined as “stable dose statin therapy,
with optional dose adjustment (within the range approved by health authority)”.
In the PCSK9 MaB group, a personalized approach was employed for blood lipid-lowering using PCSK9 MaBs. Alirocumab was utilized in 8 studies, while Evolocumab was used in 3 studies. Among the 3 studies involving Evolocumab, the control group received standard of care in 1 study and a placebo in 2 studies. Among the seven ODISSEY articles, Schwartz et al. [16, 21, 22] primarily focused on several aspects. Firstly, they examined the potential for the recurrence of ischemic cardiovascular events in patients with ACS. They aimed to determine whether Alirocumab could enhance cardiovascular outcomes in ACS patients who were already on high-intensity statins. Additionally, they investigated the impact of Alirocumab on LDL-C levels and lipoprotein(a) levels in ACS patients and explored the safety profile of Alirocumab in this patient group. Steg et al. [17] centered their study on evaluating the effect of Alirocumab on mortality in ACS patients, while Goodman et al. [18] emphasized the assessment of cardiovascular outcomes in ACS patients. Damask et al. [19] made a special effort to analyze the risk of MACEs. Hagström et al. [20] conducted a study that focused on the residual cardiovascular risk and Apolipoprotein B (ApoB) levels in ACS patients.
In terms of the study endpoints, 9 articles considered MACE as the primary endpoint, while 4 articles used any cardiovascular disease as the safety endpoint.
As per the Cochrane Risk of Bias assessment tool, 10 articles, with the exception of Li et al. [24], utilized random sequence generation and allocation concealment methods. These studies were also conducted with double-blind designs. Given that all the RCTs included in the analysis provided complete data, the risk of bias in incomplete outcome data was deemed to be low. For the remaining potential sources of bias, the risk of bias in these studies was unclear. The risk of bias assessment results are presented in Fig. 2.
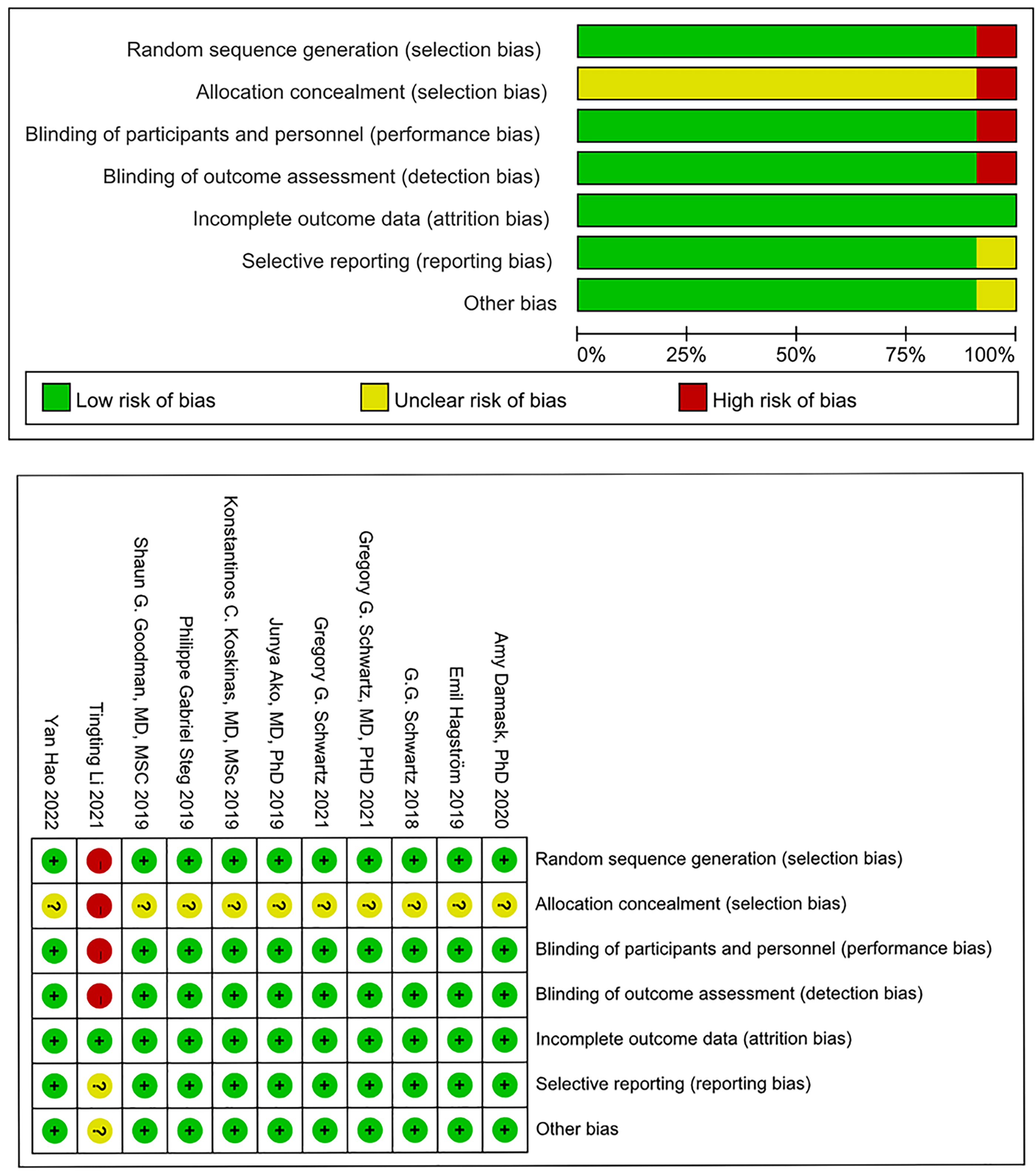
Result of risk of bias assessment.
In total, the ODISSEY studies encompassed 18,924 patients who were aged 40 years
or older and had experienced ACS within 1 to 12 months prior to their
randomization. MACEs occurred in 811 (8.25%) out of 9826 patients in the
experimental group, while 925 (9.4%) out of 9823 patients in the control group
experienced MACEs. Breaking down the individual endpoints, 786
patients (45.2%) suffered from all-cause death, 470 patients (27.1%)
experienced myocardial infarctions, 394 patients (22.7%) had strokes, and 83
patients (4.8%) faced stent thrombosis. We conducted a meta-analysis for each
MACE endpoint. Consequently, a total of 9 articles (comprising 7 ODISSEY articles
and 2 RCTs) reported data related to the all-cause mortality.
The results showed that PCSK9 MaB may reduce the risk of MACEs when compared to
the control group. Notably, the 7 ODISSEY-related articles employed varying
inclusion and exclusion criteria in the process of selecting participants,
ultimately yielding diverse outcomes. Damask et al. [19] enrolled 11,953
ACS patients with pharmacogenomic data. Hagström et al. [20]
concentrated on analyzing MACE in a cohort of 18,924 patients. Schwartz et al. [16] directed their attention towards investigating changes in lipid
levels in the same group of 18,924 patients. Additionally, Schwartz et
al. [22] initially screened 18,924 patients and ultimately included 18,490
individuals who met at least one of the following criteria: low-density
lipoprotein (LDL) cholesterol level
greater than or equal to 70 mg/dL (1.8 mmol/L), non-high-density lipoprotein (HDL) cholesterol level greater
than or equal to 100 mg/dL (2.6 mmol/L), or apolipoprotein B level at least 80
mg/dL. Schwartz et al. [21] focused on analyzing MACE in all patients
after 4 months of treatment. Steg et al. [17] focused on cardiovascular
deaths, while Goodman et al. [18] categorized patients based on
their coronary artery bypass grafting (CABG) status and
specifically enrolled ACS patients without CABG (n = 16,896). After summarizing
the diverse results, we found that, within the ODISSEY studies, PCSK9 MaB
demonstrated the potential to reduce the incidence of MACE in patients
(p
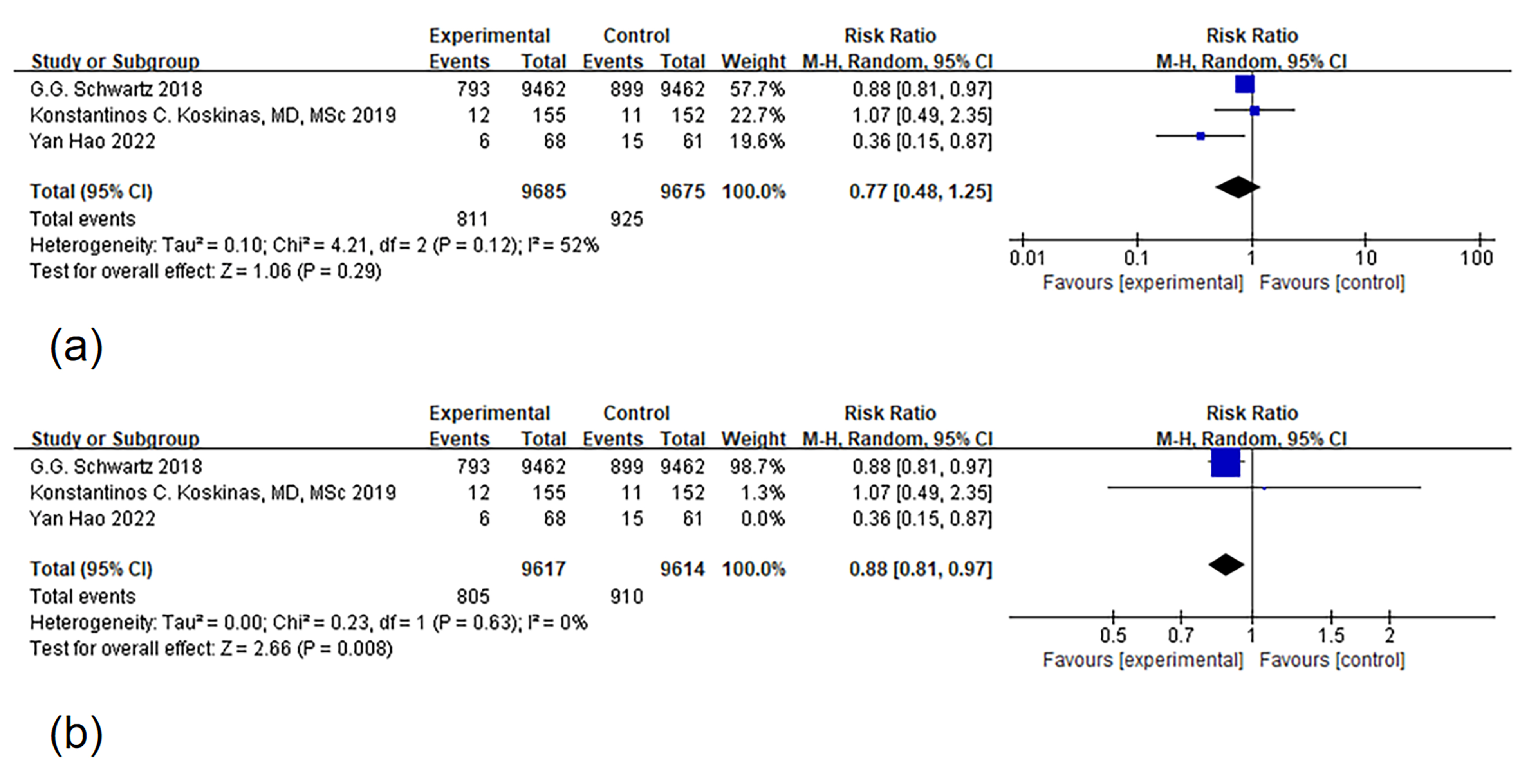
Forest plot of major adverse cardiovascular event (MACE). Notes: (a) Forest map of MACE (b) Articles were systematically excluded one by one, and after this process, two articles remained for a thorough analysis of heterogeneity. The root of the heterogeneity was eventually traced back to the study conducted by Hao [25]. Upon exclusion of this particular study, the issue of heterogeneity ceased to be a factor.
ACS recurrence was reported in 3 ODISSEY-related articles, which employed
differing inclusion and exclusion criteria for enrolling participants.
Consequently, the outcome indicators varied across these studies. We
meta-analyzed the diverse outcome data from these three articles and observed
that PCSK9 MaB could reduce the recurrence rate of ACS
(p

Forest plot of acute coronary syndrome. Abbreviation: 95% CI, 95% confidence interval.
Due to variations in the parameters measured across the five RCTs, the
standardized mean difference (SMD) was chosen as the statistical measure. When
comparing ApoB between the PCSK9 MaB group and the control group, we observed
substantial heterogeneity (I

Forest plot of ApoB. Abbreviation: ApoB, Apolipoprotein B; 95% CI, 95% confidence interval.
Both Hagström et al. [20] and Schwartz et al. [21]
conducted ODISSEY-related studies that assessed and analyzed LDL-C values.
However, Hagström et al. [20] excluded patients with apoB levels
less than 35 mg/dL. Furthermore, LDL-C data was missing for 3 patients, which had
an impact on the overall LDL-C results. Therefore, we performed a meta-analysis
that included the ODISSEY study by Schwartz et al. [21] along with four
other articles. The meta-analysis results indicated a significant decrease in
LDL-C levels within the PCSK9 MaB group (SMD [95% CI] = –2.05 [–2.61,
–1.48], p

Forest plot of LDL-C. Abbreviation: LDL-C, Low density lipoprotein cholesterol; 95% CI, 95% confidence interval.
Three RCTs reported non-HDL-C endpoints. The analysis showed a significant level
of heterogeneity (I

Forest plot of Non–HDL-C. Abbreviation: Non–HDL-C, non-high-density lipoprotein cholesterol; 95% CI, 95% confidence interval.
Both ODISSEY studies conducted by Hagström et al. [20] and
Schwartz et al. [21] examined the levels of Lipoprotein(a). Notably, no
drop-out patient data was observed in the entirety of Schwartz’s trial [21].
Consequently, these two studies, along with other four articles, were included in
the meta-analysis. A random-effects model was used. While there
was a substantial degree of heterogeneity, it did not reach statistical
significance. To ensure the robustness of the results, we systematically excluded
articles one by one, and no significant changes in the level of heterogeneity
were detected. In summary, PCSK9 MaB significantly reduced the Lipoprotein(a)
levels in ACS patients (I

Forest plot of Lipoprotein(a). Abbreviation: 95% CI, 95% confidence interval.
Four RCTs provided data on triglycerides. While there was a notable level of
heterogeneity, it did not reach statistical significance (I

Forest plot of Triglycerides. Abbreviation: 95% CI, 95% confidence interval.
Three RCTs provided data on HDL-C. Notably, no significant heterogeneity was
observed (I

Forest plot of HDL-C. Abbreviation: HDL-C, high-density lipoprotein cholesterol; 95% CI, 95% confidence interval.
To date, this study stands as the inaugural systematic review and meta-analysis aiming to assess the laboratory and clinical advantages of PCSK9 MaB to reduce lipid levels. Our findings may serve to foster a more holistic integration of PCSK9 MaB into clinical practice.
This systematic review and meta-analysis have unveiled the substantial benefits of personalized lipid-lowering treatment with PCSK9 MaB, notably in terms of lowering the risk of cardiovascular recurrence and MACEs in ACS patients when compared to conventional treatment. PCSK9 MaB is primarily synthesized in the endoplasmic reticulum. The process begins with the formation of a 75 kDa precursor, and this immature precursor comprises an N-terminal signal peptide sequence, a pre-domain, a catalytic domain, and a C-terminal domain rich in semiphotopine [18]. The binding of the pre-domain may play a role in inhibiting protease activity [26]. In ACS patients, cardiac overload and impaired blood flow contribute to MACE. Experiments have shown that lipoprotein lipase, a plasma-specific enzyme, acts as a catalyst in plasma [27], accelerating blood flow. After cleavage, the prestructure of PCSK9 MaB forms a non-covalent bond with the catalytic domain, creating a complex with mature fragments. It leaves the endoplasmic reticulum as a molecular chaperone and, following a series of modifications such as acetylation [28], is eventually secreted into the bloodstream. This process inhibits the activity of lipoprotein lipase, subsequently slowing down blood flow due to the loss of lipoprotein enzyme catalysis. PCSK9 MaB intervenes in the action mechanism of PCSK9, preserving protease activity. This blood protease can serve as a catalyst to restore normal blood flow speed and gradually enhance heart pumping function, thereby reducing the recurrence of cardiovascular events and the risk of MACE. This meta-analysis has demonstrated that PCSK9 MaB treatment effectively reduces blood lipid levels in ACS patients. PCSK9 MaB, serving as an enzyme that regulates nerve cell apoptosis, influences not only liver regeneration and nerve cell apoptosis but also the internal transformation of low-density lipoprotein receptor (LDLR). It reduces the number of LDLR in liver cells, thus impeding the clearance of LDL from the blood and leading to hypercholesterolemia [29]. Hypercholesterolemia is associated with liver-derived secreted protein that binds to the extracellular domain of LDLR and subsequently degrades LDLR within cells [30]. Studies have shown a significant positive correlation between blood PCSK9 levels and cholesterol, LDL, and triglyceride levels [31]. PCSK9 itself does not require the catalytic activity of kinases to impact LDLR transformation, and kinase activity does not guide or inhibit complex degradation [30]. As a serine protease, PCSK9 degrades LDLR, resulting in elevated blood LDL levels. Therefore, PCSK9 MaB, when used as monotherapy or in combination therapy, inhibits PCSK9 and effectively reduces LDL levels. For example, Evolocumab, an all-human IgG2 monoclonal antibody, serves as a PCSK9 inhibitor. It binds to PCSK9 and inhibits its interaction with low-density lipoprotein receptor and lipoprotein(a) receptor, thus preventing PCSK9-mediated degradation of these receptors [32]. Lipoprotein(a) is a low-density lipoprotein particle whose concentration is largely hereditary and is considered to have atherogenic, pro-inflammatory, and pro-thrombotic properties [16]. Additionally, PCSK9 MaB can reduce lipoprotein(a) concentrations by 20% to 25% [22]. Given the close connection between abnormal blood lipid parameters and a high risk of MACE, it is reasonable to expect that PCSK9 MaB can effectively lower the risk of MACE in these patients. Therefore, ACS patients receiving PCSK9 MaB may significantly benefit.
Plasma PCSK9 exists predominantly as a heterodimer (62 + 13 kDa), which is considered an active form due to its high affinity to LDL receptors (LDLR). A less active form of plasma PCSK9 with a lower molecular weight (with approximately two-fold reduced affinity to LDLR) can also be found [33]. In contrast, intracellular PCSK9 is only present in its proprotein form or as a preformed heterodimer. PCSK9 is involved both directly and indirectly in the process of atherosclerotic plaque formation [34]. PCSK9 MaB demonstrated a relatively safe profile with no significantly higher incidence of adverse events beyond cardiovascular events when compared to the control group. Previous studies have indicated that statin drugs can lead to abnormal liver function in some individuals with prolonged usage [25]. In this study, we explored this matter as well. Our findings revealed that, in contrast to a placebo, PCSK9 MaB had no greater impact on liver function. This suggests that PCSK9 MaB may offer an advantage over statins. In terms of the production of PCSK9, the PCSK9 zymogen is initially synthesized in the endoplasmic reticulum, and undergoes self-catalytic reactions either in the endoplasmic reticulum or golgiosome, leading to the cleavage and release of the propeptide. This process results in the formation of mature protease, which is immediately secreted into the bloodstream. PCSK9, through its regulation of LDLR, helps maintain the stability of plasma lipid levels, influences plasma cholesterol levels, modulates nerve cell apoptosis, and is associated with inflammatory responses [35]. As an inhibitor, PCSK9 MaB possesses various biological functions, including participation in nervous system development, regulation of nerve cell apoptosis, modulation of sodium channels, and influence on islet cell function. These functions may contribute to the restoration of impaired liver and kidney functions.
Currently, in addition to the studies mentioned above, there are several ongoing
investigations on PCSK9 MaB. For example, the EVOLVE-MI study (NCT05284747) is
assessing the clinical efficacy of PCSK9 MaB by monitoring the cumulative time to
all-cause mortality in ACS patients over a 3.5-year period, as well as the
changes in baseline LDL-C levels at 12 weeks and 52 weeks. Based on the findings
of this meta-analysis, it is anticipated that all-cause mortality will be
reduced, and there will be a significant improvement in baseline LDL-C levels.
The VICTORION-INCEPTION trial (NCT04873934) is specifically focused on comparing
the time it takes to achieve an LDL-C reduction
This study has several limitations worth noting. Firstly, certain studies exhibited substantial heterogeneity, and the source of this heterogeneity could not be effectively determined due to the limited number of included articles. Secondly, given that the majority of the studies were derived from the ODISSEY trials, the limited number of included studies, single clinical grouping, and lack of publication bias testing, further comparative investigations are required to gain a more comprehensive understanding of the causes of MACE risk.
When PCSK9 MaB is employed as a monotherapy or as part of combination therapy, ACS patients experience a reduced risk of cardiovascular recurrence events and MACEs. It is worth noting that patients at a high risk of ACS derive greater absolute benefits from PCSK9 MaB treatment. Additionally, PCSK9 MaB significantly lowers blood lipid parameters such as LDL, ApoB, and Triglycerides, without any discernible safety concerns. However, owing to the preponderance of the ODISSEY trials, the limited number of included studies, the single clinical grouping, and the absence of a publication bias analysis, further studies with more diverse clinical groupings and comparative analyses are warranted to investigate the underlying causes of MACE risk. Future meta-analyses should consider subdividing major adverse events and recurrent cardiovascular diseases within the ACS patient population. Moreover, a greater number of studies are needed to explore the specific impact of PCSK9 MaB on individual diseases.
PCSK9, proprotein convertase subtilisin/kexin type 9 inhibitor; MACEs, major adverse cardiovascular events; ACS, acute coronary syndrome; RR, risk ratio; ACVC, Acute Cardiovascular Care; EAPC, European Association of Preventive Cardiology; ESC, European Society of Cardiology; LDL-C, low density lipoprotein cholesterol.
The original contributions presented in the study are included in the article/supplementary material, further inquiries can be directed to the corresponding author.
JJZ designed the study. XYT and JJZ conducted the research. SL provided help and advice. CXL and JP analyzed the data, and all authors edited, revised the manuscript, and approved the final version. Furthermore, all authors participated in and are partially responsible for their own work.
Not applicable.
Not applicable.
1. Study on the biological network integration and regulation mechanism in the treatment of dilated cardiomyopathy with Linggui Yangxin Decoction (Approval No.: 82274427); 2. Study on the mechanism of Shenqi Yangxin Decoction to improve dilated cardiomyopathy by regulating DNA methylation involving DNMT1 to inhibit pyroptosis (Project Approval No.: BK20201141); 3. Jiangsu Provincial Natural Science Foundation Project (BK20201141).
The authors declare no conflict of interest.
Publisher’s Note: IMR Press stays neutral with regard to jurisdictional claims in published maps and institutional affiliations.